Exercise-induced bronchoconstriction (EIB) is a prominent asthma phenotype affecting an estimated 90 % of asthma patients and up to 50 % of elite athlete populations( Reference Carlsen, Anderson and Bjermer 1 ). EIB is characterised by transient airway narrowing during and/or after exercise( Reference Weiler, Anderson and Randolph 2 ) and is ascribed to airway drying leading to degranulation of inflammatory cells and release of inflammatory mediators( Reference Hallstrand 3 , Reference Hallstrand, Altemeier and Aitken 4 ). Inhaled corticosteroids and short- and long-acting β 2-agonists are effective therapies, but they are not curative and do not modify disease progression( Reference Barnes 5 ). Furthermore, inhaled corticosteroids adherence is notoriously poor and may have undesirable side effects, whereas chronic β 2-agonist use results in tolerance( Reference Barnes 5 , Reference Ramage, Lipworth and Ingram 6 ). Development of therapies that modulate asthma immunopathology without adverse side effects is therefore desirable.
One potential candidate therapy involves the long-chain n-3 PUFA, EPA and DHA( Reference Kumar, Mastana and Lindley 7 ). Dietary supplementation of n-3 PUFA increases cell membrane EPA and DHA content and reduces n-6 arachidonic acid content( Reference Calder 8 ). This subsequently reduces the synthesis of the pro-inflammatory arachidonic acid-derived eicosanoids cysteinyl leukotrienes and PG. EPA and DHA may also increase synthesis of resolvin and protectin compounds through the cyclo-oxygenase and lipoxygenase pathways which are involved in the resolution of inflammation. These mechanisms are thought to be central to the well-established anti-inflammatory effects of n-3 PUFA( Reference Calder 8 ), which provide a rationale for the use of n-3 PUFA in asthma( Reference Kumar, Mastana and Lindley 7 ). To date, however, the role of EPA and DHA in the management of asthma and EIB remains uncertain( Reference Arm, Horton and Mencia-Huerta 9 – Reference Weiler, Brannan and Randolph 14 ). Early research showed that supplementation of 5·4 g/d n-3 PUFA (3·2 g/d EPA and 2·2 g/d DHA) for 10 weeks in adults with asthma reduced leukotriene generation and neutrophil chemotactic responsiveness, but did not reduce bronchoconstriction after cycling exercise and a histamine challenge( Reference Arm, Horton and Mencia-Huerta 9 ). Similarly, supplementation of 6·0 g/d n-3 PUFA (4·0 g/d EPA and 2·0 g/d DHA) for 3 weeks in adults with asthma did not attenuate bronchoconstriction or markers of airway inflammation in response to a mannitol challenge test( Reference Brannan, Bood and Alkhabaz 12 ). Conversely, supplementation of 5·4 g/d n-3 PUFA (3·2 g/d EPA and 2·2 g/d DHA) for 3 weeks in elite athletes( Reference Mickleborough, Murray and Ionescu 10 ) and physically active asthmatic males( Reference Mickleborough, Lindley and Ionescu 11 , Reference Kumar, Mastana and Lindley 15 ) abolished EIB. Subsequent studies showed that supplementation of n-3 PUFA (3·2 g/d EPA and 2·0 g/d DHA) reduced bronchoconstriction and markers of airway inflammation after eucapnic voluntary hyperpnoea (EVH)( Reference Mickleborough and Lindley 16 ) and that n-3 PUFA 3·2 g/d EPA and 2·0 g/d DHA) was as effective as a leukotriene modifier( Reference Tecklenburg-Lund, Mickleborough and Turner 17 ).
Given the lack of reported risk in taking n-3 PUFA, the American Thoracic Society/European Respiratory Society (ATS/ERS) guidelines suggest that the latter studies offer some support for the consumption of n-3 PUFA by interested individuals with EIB( Reference Mickleborough, Murray and Ionescu 10 , Reference Mickleborough, Lindley and Ionescu 11 , Reference Tecklenburg-Lund, Mickleborough and Turner 17 ). In contrast however, recent practice parameter( Reference Weiler, Brannan and Randolph 14 ) cautions the recommendation for n-3 PUFA use in EIB based on more recent findings( Reference Brannan, Bood and Alkhabaz 12 , Reference Price, Hull and Howatson 18 ). When considering the practicalities associated with n-3 PUFA supplementation for asthma management, previous studies have used doses of 5·2–6·0 g/d. Such doses require individuals to ingest between eight and twenty capsules of commercial fish oil daily, which has implications for cost, compliance and gastrointestinal discomfort( Reference Burns, Halabi and Clamon 19 , Reference Walser and Stebbins 20 ). In a single-blind study an alternative flavoured beverage delivery was investigated (3·0 g/d EPA, 3·0 g/d DHA and 30 µg of vitamin D3) but failed to show any attenuation in hyperpnoea-induced bronchoconstriction (HIB) or markers of airway inflammation (fraction of exhaled nitric oxide (FENO), urinary 9α, 11β PGF2 and cysteinyl leukotriene E4)( Reference Price, Hull and Howatson 18 ). However, no measure of compliance to the treatment was made and participants included had mild asthma or did not have a physician diagnosis of asthma. It is therefore pertinent to establish if lower doses of n-3 PUFA are effective in reducing EIB. With respect to the simulation of EIB under laboratory conditions, an EVH challenge causes a highly reproducible HIB (a surrogate for EIB) in adults with asthma( Reference Williams, Johnson and Hunter 21 ) which makes this an attractive challenge test to evaluate the effects of n-3 PUFA treatments on airway hyperresponsiveness. Thus, the aim of the current study was to compare the effects of a 6·2 g/d n-3 PUFA dose with a half dose of 3·1 g/d n-3 PUFA on HIB and markers of airway inflammation in adults with asthma. Provisional data from the study was previously part published in abstract form( Reference Williams, Hunter and Johnson 22 ).
Methods
Participants
In all, sixteen non-smoking, recreationally active men (completing ≥6 h of endurance exercise per week) provided written, informed consent to participate in the study (Table 1). Eight participants formed a HIB group and eight formed a control group. Inclusion criteria for the HIB group were: physician diagnosis of asthma, a baseline forced expiratory volume in 1 s (FEV1) >65 % of predicted( Reference Argyros, Roach and Hurwitz 23 ) and a ≥10 % fall in FEV1 following initial EVH screening( Reference Anderson, Argyros and Magnussen 24 , Reference Parsons, Hallstrand and Mastronarde 25 ). The HIB group were on steps 1–3 of the stepwise approach to asthma control, indicating well-controlled asthma using either reliever medication (short-acting β 2-agonsit) alone or in combination with controller medication (low-dose inhaled corticosteroid and/or long-acting β 2-agonist( Reference Boulet, FitzGerald and Reddel 26 ) (Table 1). Inclusion criteria for the control group were: a baseline FEV1 >65 % of predicted and a <10 % fall in FEV1 following initial EVH screening. Participants avoided exercise for 24 h before an EVH test, and the HIB group ceased their medication as previously described( Reference Williams, Johnson and Hunter 21 , Reference Anderson, Argyros and Magnussen 24 ). On EVH test days, participants abstained from caffeine and alcohol and arrived at the laboratory >2 h post-prandial( Reference Duffy and Phillips 27 , Reference Vally and Thompson 28 ). Participants were instructed to abstain from consumption of n-3 PUFA supplements and eat no more than two oily fish meals per week for 3 weeks before the study and throughout the study( Reference Mickleborough, Lindley and Ionescu 11 ). Participants were free from acute upper respiratory tract infections throughout the study.
Table 1 Individual anthropometric data, baseline pulmonary function and medication (Individual values; mean values and standard deviations; percentage predicted)

FVC, forced vital capacity; FEV1, forced expiratory volume in 1 s; HIB, hyperpnoea-induced bronchoconstriction; S, salbutamol; BUD, budesonide; FORM, formoterol; BEC, beclomethasone.
*Difference between the HIB and control groups (P=0·017).
Experimental design and protocol
This study was conducted in accordance with the Declaration of Helsinki and all procedures were approved by the Nottingham Trent University Human Ethics Committee (approval no. 186; Clinical trial no. ISRCTN80857707). The study adopted a counter balanced, double-blind, placebo-controlled crossover design over 14 consecutive weeks (Fig. 1).

Fig. 1 Participant flow diagram. HIB, hyperpnoea-induced bronchoconstriction; CTRL, controls; GI, gastrointestinal.
Participants were randomised (block randomisation) to receive three 21 d treatments each separated by a 14-d washout period. All treatments involved daily oral consumption of eight capsules (Croda International Plc). Four capsules were taken in the morning and four in the afternoon with a recommendation to take with food. The three treatments were: 6·2 g/d n-3 PUFA (3·7 g EPA and 2·5 g DHA), 3·1 g/d n-3 PUFA (1·8 g EPA and 1·3 g DHA) (INCROMEGATM TG4030; Croda International Plc) and placebo (CRODAMOLTM GTCC medium chain TAG; Croda International Plc) (Table 2). CRODAMOLTM GTCC was chosen as it is readily oxidised in the liver so has little impact on human health-related biomarkers( Reference Yusof, Miles and Calder 29 , Reference Bahal, Romansky and Alvarez 30 ). Measurements were taken at days 0 and 21 of each treatment period. The 6·2 g/d n-3 PUFA dose was comprised of four n-3 PUFA capsules in the morning and four n-3 PUFA capsules in the afternoon. To ensure an equal number of capsules were taken the 3·1 g/d n-3 PUFA comprised of two n-3 PUFA capsules and two placebo capsules in the morning and two n-3 PUFA capsules and two placebo capsules in the afternoon. All capsules (placebo and n-3 PUFA) were identical in appearance.
Table 2 Fatty acid composition (% total fatty acids) of the n-3 PUFA (INCROMEGATM TG4030) and placebo (CRODAMOLTM GTCC, medium-chain TAG) treatmentsFootnote *
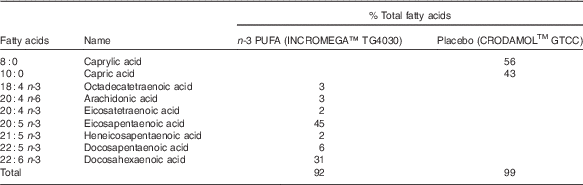
* Individual fatty acids making up ≥1 % are shown.
Measurement of pulmonary function, eucapnic voluntary hyperpnoea and fraction of exhaled nitric oxide
The EVH test was undertaken at days 0 and 21 of each treatment and comprised 6 min of breathing dry gas at a target minute ventilation (
$\dot{V}_{E} $
) of 85 % of the predicted maximal voluntary ventilation (MVV) (30×baseline FEV1). Pulmonary function (forced vital capacity (FVC), FEV1, peak expiratory flow (PEF) and forced expiratory flow 25–75 % (FEF25–75 %)) was assessed according to ATS/ERS guidelines(
Reference Miller, Hankinson and Brusasco
31
) in triplicate at baseline and in duplicate at 3, 6, 16, 20 and 30 min after EVH, as previously described. The highest values recorded were used for analyses. Baseline FENO was measured (NIOX MINO; Aerocrine) according to ATS/ERS guidelines(
Reference Dweik, Boggs and Erzurum
32
) in the HIB group only, as it is elevated in asthma patients but not in healthy controls(
Reference Alving, Weitzberg and Lundberg
33
).
Urinary 9α, 11β-PGF2 analysis
Participants provided a urine sample at baseline and at 12, 60 and 90 min after EVH. Urinary concentration of 9α, 11β-PGF2, a metabolite of PGD2, was subsequently determined by ELISA (Caymen Chemicals) and standardised for urinary creatinine concentration (ABX Pentra 400; Horiba) based on a kinetic method using alkaline picrate (Jaffe method)( Reference Junge, Wilke and Halabi 34 ). The corrected 9α, 11β-PGF2 was expressed as ng/mmol creatinine. The inter- and intra-assay CV was <15 %. Two control participants and one HIB participant had samples at day 0 below the limit of detection for urinary 9α, 11β-PGF2 (5 pg/ml) and were subsequently excluded from analysis.
Neutrophil phospholipid fatty acid analysis
The neutrophil phospholipid fatty acid composition was assessed as a measure of compliance to the treatments in addition to count of capsules returned after each treatment. Neutrophil cells were isolated from 20 ml of whole venous blood (drawn at baseline from an antecubital vein) through a three-step purification protocol that consisted of dextran sedimentation (Fisher Scientific), hypotonic lysis and Ficoll–Paque sedimentation (GE Healthcare)( Reference Nauseef 35 ). This method achieved up to 98 % of pure neutrophils which were stored at −80°C under N until extraction of phospholipids using previously described methods( Reference Bligh and Dyer 36 ). Fatty acid composition was analysed by GC as previously described( Reference Jackson, Bateman and Yaqoob 37 ). To identify the fatty acid methyl esters (FAME), retention times were compared against known standards, Supelco 37 component FAME mix and PUFA-3 menhaden oil (Sigma). EPA, DHA, arachidonic acid and linoleic acid were expressed as a percentage of total fatty acids.
Statistical analysis
The average minimum perceptible improvement in FEV1 in adults with asthma is 230 ml( Reference Santanello, Zhang and Seidenberg 38 ), whereas the within participant sd for the fall in FEV1 after EVH is 100 ml( Reference Williams, Johnson and Hunter 21 ). A priori sample size calculation revealed that with power=0·90 and α=0·05, a sample size of seven in the HIB group would be required to detect a 230 ml improvement in the fall in FEV1 after EVH.
Data were analysed using SPSS. Following assessment for normality (Shapiro–Wilk test, skewness and kurtosis), data were analysed using repeated measures ANOVA and Bonferroni adjusted paired t tests. Statistical significance was set at P<0·05. Data presented are mean values and standard deviations unless otherwise stated. Within treatment percentage differences (from day 0 to day 21) for the fall in FEV1 following EVH were calculated as: ((% fall day 0−% fall day 21)/% fall day 0)×100. For the HIB group, the individual percentage protection afforded by the n-3 PUFA treatments compared with placebo was calculated for the % fall in FEV1 following EVH as: ((% fall day 21 placebo−% fall day 21 treatment)/% fall day 21 placebo)×100. In the HIB group, the overall severity of HIB was determined by calculating the AUC for % fall in FEV1 after EVH (AUC0–30) using the trapezoidal rule.
Results
Pulmonary function and ventilation rate during eucapnic voluntary hyperpnoea
Baseline FEV1 was lower in the HIB group (3·75 (sd 0·81) litres) than the control group (4·63 (sd 0·37) litres) (P=0·017) and FVC tended to be lower in the HIB group (4·66 (sd 0·06) litres) than the control group (5·26 (sd 0·39) litres) (P=0·051). There were no within-group differences in FEV1 or FVC measured at day 0 between the three treatments.
As expected, at day 0 there was a greater peak fall in FEV1 after EVH in the HIB group (pooled data: −29 (sd 17) %) than the control group (pooled data: −3 (sd 2) %) (P=0·001). In the control group, there was no effect of treatment or day on the peak fall in FEV1 after EVH. In the HIB group, there was a treatment×day interaction for the peak fall in FEV1 after EVH (P=0·011). Further analyses revealed an effect of treatment for the peak fall in FEV1 after EVH at day 21 (P=0·001). Specifically, the peak fall in FEV1 after EVH was reduced by 34 (sd 14) % (−690 (sd 460) ml) after 6·2 g/d n-3 PUFA (mean difference=310 (sd 150) ml, 95 % CI 185, 432 ml, P=0·001, effect size=0·70), and by 30 (sd 11) % (−700 (sd 420) ml) after 3·1 g/d n-3 PUFA (mean difference=270 (sd 120) ml, 95 % CI 170, 377 ml, P=0·001, effect size=0·58). The reduced peak falls in FEV1 after 6·2 and 3·1 g/d n-3 PUFA were not different (P=0·834) (Fig. 2, Table 3). The percentage protection afforded by the n-3 PUFA treatments compared with placebo is shown in Table 3. The peak fall in FEV1 was unchanged from day 0 to day 21 of placebo.

Fig. 2 Peak falls in forced expiratory volume in 1 s (FEV1) before and after placebo (a), 6·2 g/d n-3 PUFA (b), and 3·1 g/d n-3 PUFA (c) in the hyperpnoea-induced bronchoconstriction (HIB) group (n 8). Values are means, and standard deviations represented by vertical bars and identical symbols represent the same HIB participant. * Day 0 v. day 21 (P=0·001).
Table 3 Individual peak % fall in forced expiratory volume in 1 s (FEV1) at days 0 and 21 of each treatment and percentage protection afforded by n-3 PUFA in the hyperpnoea-induced bronchoconstriction (HIB) group

* Percentage protection afforded by the n-3 PUFA treatments calculated as: ((% fall day 21 placebo−% fall day 21 treatment)/% fall day 21 placebo)×100.
Fig. 3 shows the percentage change in FEV1 during 30 min recovery after EVH in the HIB group. At day 0, the percentage change in FEV1 during recovery was not different between treatments. At day 21, the percentage change in FEV1 was reduced for up to 20 and 30 min recovery after 6·2 and 3·1 g/d n-3 PUFA, respectively. Furthermore, compared with placebo at day 21, the percentage change in FEV1 was lower for up to 6 and 20 min recovery after 6·2 and 3·1 g/d n-3 PUFA, respectively.

Fig. 3 Percentage change in forced expiratory volume in 1 s (FEV1) after eucapnic voluntary hyperpnoea (EVH) in participants with hyperpnoea-induced bronchoconstriction (HIB). A 10 % fall in FEV1 (shown by the ) is diagnostic of HIB.
, Day 0 of placebo treatment;
, day 21 of placebo treatment;
, day 0 of 6·2 g/d n-3 PUFA treatment;
, day 21 of 6·2 g/d n-3 PUFA;
, day 0 of 3·1 g/d n-3 PUFA;
, day 21 of 3·1 g/d n-3 PUFA. Mean values with unlike letters were significantly different: a, 6·2 g/d n-3 PUFA v. placebo; b, 3·1 g/d n-3 PUFA v. placebo; c, day 0 v. day 21 of 6·2 g/d n-3 PUFA; d, day 0 v. day 21 of 3·1 g/d n-3 PUFA. P<0·05, * P<0·01, ** P<0·001.
In the HIB group, there was a treatment×day interaction for AUC0–30 (P=0·004). Further analysis revealed that at day 21 the AUC0–30 was reduced after 6·2 g/d (−415 (sd 382), P=0·002) and 3·1 g/d (−398 (sd 399), P=0·001) n-3 PUFA compared with placebo (−595 (sd 424)). The AUC0–30 at day 21 of 6·2 and 3·1 g/d n-3 PUFA was not different (P=0·751).
Consistent with our previous findings( Reference Williams, Johnson and Hunter 21 , Reference Williams, Johnson and Shaw 39 ) the peak fall in FEV1 after EVH in the HIB group was reproducible with no differences occurring between day 0 of the three treatments (within participant CV=7 (sd 4) %; measurement error=84 ml; reproducibility=231 ml; smallest meaningful change=115 ml). The peak fall in FVC showed similar outcomes. At day 0 of the three treatments the peak fall in FVC was greater in the HIB group (pooled data: −992 (sd 604) ml; −21 (sd 15) %) than the control group (pooled data: −140 (sd 90) ml; −3 (sd 2) %) (P=0·004). In the control group, the peak fall in FVC was unchanged after placebo and both doses of n-3 PUFA. The peak fall in FVC after EVH at day 0 was reduced by 30 (sd 21) % after 6·2 g/d n-3 PUFA and by 29 (sd 24) % after 3·1 g/d n-3 PUFA but was unchanged after placebo in the HIB group. The reduced peak fall in FVC after 6·2 and 3·1 g/d n-3 PUFA was not different (P=0·847). In the HIB group, the peak fall in PEF was unchanged after placebo and both n-3 PUFA treatments (pooled data: −2·52 (sd 1·68) litre/s). In the HIB group, the peak fall in FEF25–75 % was reduced from day 0 (−1·55 (sd 0·37) litre/s) to day 21 (−1·24 (sd 0·45) litre/s) of 6·2 g/d n-3 PUFA (mean difference=0·31 (sd 0·23) litre/s, 95 % CI 0·12, 0·50 litre/s, P=0·006, effect size=0·77). In the HIB group, the peak fall in FEF25–75 % was unchanged after 3·1 g/d n-3 PUFA and placebo in the HIB group, and after all treatments in the control group (P>0·05).
Minute ventilation achieved during each of the six EVH trials did not differ in the HIB group (P>0·05; between trial CV=0·23) (pooled data: 109·5 (sd 21·4) litre/min; 78 (sd 18) % of MVV target) which was less compared with the control group (P=0·001; between trial CV=0·09) (pooled data: 133·4 (sd 10·8) litre/min; 60 (sd 6) % of MVV target).
Fraction of exhaled nitric oxide
In the HIB group, there was a treatment×day interaction for FENO (P=0·004). After 6·2 g/d n-3 PUFA, FENO was reduced by 24 % from day 0 (48 (sd 33) parts per billion (ppb)) to day 21 (35 (sd 28) ppb) (mean difference=13 (sd 12) ppb, 95 % CI 3, 24 ppb, P=0·020, effect size=0·41). Similarly, after 3·1 g/d n-3 PUFA, FENO was reduced by 31 % from day 0 (49 (sd 33) ppb) to day 21 (34 (sd 28) ppb) (mean difference=15 (sd 14) ppb, 95 % CI 4, 27 ppb, P=0·018, effect size=0·46) (Fig. 4). The reduced FENO after 6·2 and 3·1 g/d n-3 PUFA was not different (P=0·491) (Fig. 4). FENO remained unchanged from day 0 (47 (sd 26) ppb) to day 21 (47 (sd 27) ppb) of placebo.

Fig. 4 Baseline fraction of exhaled nitric oxide (FENO) before and after placebo (a), 6·2 g/d n-3 PUFA (b) and 3·1 g/d n-3 PUFA (c) in the hyperpnoea-induced bronchoconstriction (HIB) group (n 8). Values are means, and standard deviations represented by vertical bars and identical symbols represent the same HIB participant. * Day 0 v. day 21 (P<0·05) ppb, Parts per billion.
Urinary 9α, 11β PGF2
Baseline urinary 9α, 11β PGF2 did not differ between the HIB group (day 0 pooled data: 40·85 (sd 21·49) ng/mmol creatinine) and the control group (day 0 pooled data: 27·05 (sd 14·01) ng/mmol creatinine) (P=0·133). There were no within-group differences in urinary 9α, 11β PGF2 measured before EVH at days 0 and 21 of the three treatments.
At day 0, urinary 9α, 11β PGF2 increased by 31·53 (sd 22·77) ng/mmol creatinine (pooled data) after EVH in the HIB group (P=0·030) but not in the control group (pooled data: 11·11 (sd 16·30) ng/mmol creatinine). At day 0, changes in 9α, 11β PGF2 after EVH were different between groups (P=0·003). In the HIB group, subsequent analyses revealed an effect of treatment on the peak increase in 9α, 11β PGF2 after EVH at day 21 (P=0·014). Specifically, the peak increase in 9α, 11β PGF2 after EVH was reduced after 6·2 g/d n-3 PUFA (mean difference=11·75 (sd 7·42) ng/mmol creatinine, 95 % CI 5·54, 17·96 ng/mmol creatinine, P=0·009, effect size=0·50) and 3·1 g/d n-3 PUFA (mean difference=12·86 (sd 14·90) ng/mmol creatinine, 95 % CI 0·40, 25·33 ng/mmol creatinine, P=0·041, effect size=0·40). The reduced peak increase in 9α, 11β PGF2 after EVH following 6·2 and 3·1 g/d n-3 PUFA was not different (P=0·377) (Fig. 5). There was no effect of placebo on the increase in urinary 9α, 11β PGF2 after EVH.

Fig. 5 Peak increase in urinary 9α, 11β PGF2 after eucapnic voluntary hyperpnoea (EVH) in the hyperpnoea-induced bronchoconstriction group (n 8). Values are means, and standard deviations represented by vertical bars. and
, Day 0 and day 21, respectively, of the treatment. * Day 0 v. day 21 (P<0·05).
Compliance assessment – neutrophil phospholipid fatty acid content, and capsule counts
Good compliance to all treatments was shown from the capsule counts in both the HIB and control groups (Table 4). Further compliance evidence was provided from the neutrophil phospholipid fatty acid content. In the HIB group, 6·2 g/d n-3 PUFA increased EPA (P=0·002) and DHA (P=0·045) content, and reduced arachidonic acid (P=0·005). In the HIB group, 3·1 g/d n-3 PUFA increased EPA content (P=0·018) and reduced arachidonic acid (P=0·009); there was a trend for an increase in DHA content (P=0·074). In the HIB group, linoleic acid content was unchanged after 6·2 and 3·1 g/d n-3 PUFA. Phospholipid fatty acid content was unchanged after placebo in both groups. In the control group, 6·2 g/d n-3 PUFA tended to increase EPA (P=0·054) and DHA content (P=0·087), whereas 3·1 g/d n-3 PUFA increased DHA content (P=0·007) and reduced arachidonic acid (P=0·038); EPA content was unchanged (Table 4).
Table 4 Treatment adherence (%) based on capsule count, and fatty acid composition of neutrophil extracts (%total fatty acids) at days 0 and 21 of each treatment in hyperpnoea-induced bronchoconstriction (HIB) and control groups (Mean values and standard deviations)

Difference between day 0 and day 21 within treatment: * P<0·05, ** P<0·01.
Discussion
Previous research has shown that high doses of n-3 PUFA (5·2–5·4 g/d) reduce the severity of HIB and EIB( Reference Mickleborough, Murray and Ionescu 10 , Reference Mickleborough, Lindley and Ionescu 11 , Reference Kumar, Mastana and Lindley 15 – Reference Tecklenburg-Lund, Mickleborough and Turner 17 ). The present study demonstrates that a lower dose of 3·1 g/d n-3 PUFA is equally effective in reducing HIB in adult men with asthma. The 6·2 and 3·1 g/d n-3 PUFA treatments also resulted in similar reductions in baseline FENO, and comparable suppression of urinary 9α, 11β-PGF2 after EVH. These findings suggest that 3·1 g/d n-3 PUFA could be used as an adjunct therapy for physically active adults with asthma and EIB. The percentage protection afforded by 6·2 g/d n-3 PUFA (35 (sd 14) %) and 3·1 g/d n-3 PUFA (33 (sd 12) %) highlights the efficacy of n-3 PUFA as an adjunct therapy. The percentage protection afforded by n-3 PUFA is comparable with montelukast (50 mg twice daily)( Reference Reiss, Hill and Harman 40 ) but less than the 60–70 % protection afforded by short-acting β 2-agonists procaterol (10 micrograms/inhalation) and albuterol (90 micrograms/inhalation)( Reference Shapiro, Kemp and DeJong 41 ). Long-acting β 2-agonists also provide effective treatment for EIB( Reference Nelson, Strauss and Skowronski 42 – Reference Bronsky, Yegen and Yeh 44 ), although chronic treatment with both long- and short-acting β 2-agonists can result in tolerance( Reference Cheung, Timmers and Zwinderman 45 , Reference Simons, Gerstner and Cheang 46 ). Furthermore, compliance to the lower 3·1 g/d n-3 PUFA dose may be improved due to reduced capsule numbers, reduce cost and risk of gastrointestinal distress, which is commonly associated with bloating, and stomach upset. Indeed, two participants withdrew from the study when on the 6·2 g/d n-3 PUFA treatment due to gastrointestinal distress, whereas full compliance and no participant withdrawals (capsule counts) was observed with 3·1 g/d n-3 PUFA.
The EVH test is an indirect bronchial provocation test that is a suitable objective surrogate for identifying EIB( Reference Anderson, Argyros and Magnussen 24 ). We have previously reported that the EVH protocol used in the present study elicits a highly reproducible fall in FEV1 ( Reference Williams, Johnson and Hunter 21 , Reference Williams, Johnson and Shaw 39 ) which is crucial when assessing treatment efficacy. The approximately 290 ml (32 %) decrease in the post-EVH fall in FEV1 after 6·2 and 3·1 g/d n-3 PUFA supplementation exceeds the minimum perceptible change of 230 ml( Reference Santanello, Zhang and Seidenberg 38 ) and is therefore clinically relevant. Our findings are in agreement with previous studies showing an attenuation of HIB( Reference Tecklenburg-Lund, Mickleborough and Turner 17 ) and EIB( Reference Mickleborough, Lindley and Ionescu 11 ) after n-3 PUFA supplementation in adults with asthma. These findings collectively support the ATS/ERS guidance( Reference Parsons, Hallstrand and Mastronarde 25 ) suggesting n-3 PUFA supplementation could be of benefit in individuals with EIB. Our findings are also in broad agreement with work on n-3 PUFA supplementation in elite athletes with EIB( Reference Mickleborough, Murray and Ionescu 10 ), and asthmatic patients with EIB and HIB( Reference Mickleborough, Lindley and Ionescu 11 , Reference Kumar, Mastana and Lindley 15 – Reference Tecklenburg-Lund, Mickleborough and Turner 17 ). An interesting difference is that previous work showed abolition of EIB after n-3 PUFA supplementation( Reference Mickleborough, Murray and Ionescu 10 ), whereas in the present study only three participants became non-diagnostic (Fig. 2). This may be partly due to inter-study differences in the severity of EIB: the peak fall in FEV1 in the present study (−30 %) was approximately 50 % greater than that reported in previous n-3 PUFA supplementation studies demonstrating a reduction in EIB/HIB( Reference Mickleborough, Lindley and Ionescu 11 , Reference Kumar, Mastana and Lindley 15 – Reference Tecklenburg-Lund, Mickleborough and Turner 17 ).
Unlike the rather consistent finding that pharmacological therapy reduces EIB(
Reference Nelson, Strauss and Skowronski
42
–
Reference Bronsky, Yegen and Yeh
44
), the efficacy of n-3 PUFA remains controversial. Our findings contrast those of Arm et al.(
Reference Arm, Horton and Mencia-Huerta
9
) and Brannan et al.(
Reference Brannan, Bood and Alkhabaz
12
) who despite using relatively high n-3 PUFA doses (5·4–6·0 g/d), reported no change in bronchial hyperresponsiveness to inhaled histamine and mannitol, respectively. Arm et al.(
Reference Arm, Horton and Mencia-Huerta
9
) reported no change in airway resistance (fall in FEV1 was not measured) after a cycling exercise challenge, although their data are confounded by: (1) the suggestion that low-grade, non-pharmaceutical n-3 PUFA was used(
Reference Mickleborough and Rundell
47
); (2) low statistical power due to the small number of participants (n 6) performing the exercise; (3) exercise being performed at ambient temperature and humidity, which may result in insufficient environmental stress; and (4) the low exercise intensity (60–130 W) which likely (data not measured/reported) elicited only a modest increase in
$\dot{V}_{E} $
. The efficacy of n-3 PUFA supplementation may also partly depend on the choice of bronchial provocation test. Direct bronchial provocation tests such as inhaled histamine act directly on receptors on the airway smooth muscle causing contraction(
Reference Brannan and Lougheed
48
) and may not, therefore, fully reveal the potential anti-inflammatory effects of n-3 PUFA. Indirect bronchial provocation tests such as mannitol and EVH both alter the tonicity and volume of the airway surface liquid thereby promoting the release of bronchoconstrictive inflammatory mediators(
Reference O’Sullivan, Roquet and Dahlen
49
,
Reference Brannan, Gulliksson and Anderson
50
). However, mannitol and EVH may exert different levels of osmotic and mechanical stress on the airways. Specifically, mannitol induces dehydration stress primarily in the proximal airways(
Reference Brannan, Gulliksson and Anderson
50
,
Reference Daviskas, Anderson and Brannan
51
), whereas the high
$\dot{V}_{E} $
during EVH results in the dehydration stress being extended to peripheral airways(
Reference Daviskas, Anderson and Gonda
52
) which are increasingly recruited to heat and humidify inspired air. Furthermore, EVH also elicits airflow-induced shear stress and high transepithelial pressure gradients(
Reference Kippelen, Fitch and Anderson
53
). These differences may predispose the airway epithelium to greater damage after EVH than mannitol; indeed, EVH tends to result in greater increases in urinary Clara cell protein 16 concentration, a marker of airway epithelial damage(
Reference Kippelen, Tufvesson and Ali
54
,
Reference Romberg, Bjermer and Tufvesson
55
). This is significant because n-3 PUFA supplementation attenuates the increase in urinary Clara cell protein concentration after EVH, which suggests that reduced HIB may be partly due to reduced airway epithelial damage(
Reference Mickleborough, Vaughn and Shei
56
). Differences in the intensity of the stimulus to the airways may thus explain the divergent effects of n-3 PUFA supplementation on bronchial hyperresponsiveness to EVH and mannitol.
Recently, Price et al.( Reference Price, Hull and Howatson 18 ) reported no change in the severity of HIB or markers of airway inflammation (FENO, urinary 9α, 11β PGF2 and cysteinyl leukotriene E4) in recreationally active adults supplemented daily with a combined treatment of 30 µg vitamin D3 with n-3 PUFA (3·0 g EPA and 2·0 g DHA). Unfortunately, objective treatment compliance measures (e.g. neutrophil phospholipid DHA and EPA content) were not taken in this study. The fall in FEV1 after EVH was also modest (approximately 16 %) compared with the approximately 30 % fall observed at day 0 in the present study, and six from ten of the participants did not have physician diagnosed asthma (and consequently no asthma medication). Two participants in Price et al.( Reference Price, Hull and Howatson 18 ) also became non-diagnostic for HIB after the placebo treatment. It may be that n-3 PUFA supplementation is thus more effective in individuals with diagnosed asthma and more severe HIB, as observed in the present study.
The increase in urinary 9α, 11β-PGF2 after EVH at day 0 in the HIB group is similar in magnitude to that reported previously in adults with asthma performing exercise or EVH( Reference Mickleborough, Murray and Ionescu 10 , Reference Simpson, Bood and Anderson 57 ). The reduced increase in 9α, 11β-PGF2 after EVH was comparable after 6·2 and 3·1 g/d n-3 PUFA supplementation. Previous work also reports a reduced increase in 9α, 11β-PGF2 after EVH following n-3 PUFA supplementation( Reference Mickleborough, Murray and Ionescu 10 ). The increased EPA and DHA cell membrane content following n-3 PUFA supplementation is likely to result in dual inhibition of arachidonic acid-dependent cyclo-oxygenase and 5-lipoxygenase pathways which are responsible for eicosanoid generation( Reference Kumar, Mastana and Lindley 7 ). This may explain the decrease in 9α, 11β-PGF2, a metabolite of the arachidonic acid-derived pro-inflammatory eicosanoid PGD2. The osmotic changes in the airways following EVH results in mast cell activation and release of PGD2 during bronchoconstriction( Reference O’Sullivan, Roquet and Dahlen 49 , Reference Brannan, Gulliksson and Anderson 50 ). Suppression of mast cell activation, and reduced eicosanoid generation, may thus partially explain reduced HIB after n-3 PUFA supplementation.
The comparable reduction in baseline FENO after 6·2 and 3·1 g/d n-3 PUFA supplementation concurs with previous work( Reference Tecklenburg-Lund, Mickleborough and Turner 17 , Reference Schubert, Kitz and Beermann 58 ) and is indicative of reduced baseline eosinophilic airway inflammation. Increased FENO can also result from elevated expression of inducible nitric oxide synthase in T-cells, macrophages, airway epithelial cells, and other inflammatory cells within the airways( Reference Hamid, Springall and Polak 59 , Reference Robbins, Springall and Warren 60 ). Elevated expression of inducible nitric oxide synthase can be induced by certain pro-inflammatory cytokines such as TNF-α ( Reference Hamid, Springall and Polak 59 , Reference Robbins, Springall and Warren 60 ) probably via the activation of NF-κB transcription factor( Reference Barnes 61 ). The n-3 and n-6 PUFA modulate NF-κB transcription factor activation( Reference Schmitz and Ecker 62 ) and presumably therefore TNF-α expression, which may explain the observed fall in FENO after n-3 PUFA supplementation in the present study. Support for this argument comes from work showing that 5·4 g/d n-3 PUFA suppresses circulating plasma TNF-α in athletes with EIB( Reference Mickleborough, Murray and Ionescu 10 ). The comparable reduction in both baseline FENO and the post-EVH increase in 9α, 11β-PGF2 after 6·2 and 3·1 g/d n-3 PUFA supplementation suggests that both doses increased the n-3 PUFA content of the phospholipid bilayer of cell membranes to influence the inflammatory response. Furthermore, and consistent with previous reports( Reference Tecklenburg-Lund, Mickleborough and Turner 17 , Reference Mickleborough, Vaughn and Shei 56 ), the lower FENO observed after n-3 PUFA supplementation in the present study may have resulted from an increase in airway pH, which is considered a determinant of FENO and airway inflammation( Reference Accordino, Visentin and Bordin 63 ).
Limitations of the current study include no run-in period to assess habitual n-3 PUFA intake, the exclusion of females, the comparatively low participant numbers, and the wide range of asthma phenotypes suggested by the heterogeneous fall in FEV1 and large variation in inflammatory markers after EVH. However, our cohort provides evidence for the use of n-3 PUFA in more severe HIB than previous literature( Reference Mickleborough, Murray and Ionescu 10 , Reference Mickleborough, Lindley and Ionescu 11 ). Future work may explore whether particular asthma phenotypes and HIB/EIB severity respond preferentially to n-3 PUFA supplementation. Furthermore, given the existing inter-study differences regarding the efficacy of n-3 PUFA for the management of EIB, further studies using larger sample sizes are warranted.
In conclusion, 3·1 g/d n-3 PUFA supplementation effectively reduced HIB in men with asthma to a similar extent as a higher dose. Lower doses of n-3 PUFA thus represent a potentially beneficial adjunct treatment for adults with asthma and EIB, whilst also reducing the burden of cost, compliance and potential gastrointestinal distress. Further studies are needed to elucidate if the bronchial provocation test used and the severity of asthma and HIB/EIB affect the degree of protection afforded by n-3 PUFA supplementation.
Acknowledgements
In-kind support of the n-3 PUFA and placebo supplements was provided by Croda Healthcare Ltd (Croda International Plc, Cowick Hall, Snaith, Goole, East Yorkshire, DN14 9AA, UK). The company was not involved in the design or implementation of the study.
N. C. W., K. A. H., D. E. S., G. R. S. and M. A. J. designed the research; N. C. W., K. A. H., G. R. S. and M. A. J. conducted research; N. C. W. and K. G. J. provided essential reagents and conducted analysis of urine and blood samples; N. C. W., G. R. S. and M. A. J analysed data. N. C. W., K. A. H., G. R. S. and M. A. J. wrote paper; N. C. W., K. A. H., D. E. S., K. G. J., G. R. S. and M. A. J. contributed to reviewing and approval of the final manuscript.
None of the authors has any conflicts of interest to declare.