Book contents
- Frontmatter
- Contents
- Preface
- 1 Target: A Useful Model
- 2 Methods of Biochemical Systems Theory
- 3 A Model of Citric Acid Production in the Mold Aspergillus niger
- 4 Optimization Methods
- 5 Optimization of Biochemical Systems
- 6 Optimization of Citric Acid Production in Aspergillus niger
- 7 Maximization of Ethanol Production in Saccharomyces cerevisiae
- 8 Conclusions
- Author Index
- Subject Index
- Plate section
- References
7 - Maximization of Ethanol Production in Saccharomyces cerevisiae
Published online by Cambridge University Press: 28 July 2009
- Frontmatter
- Contents
- Preface
- 1 Target: A Useful Model
- 2 Methods of Biochemical Systems Theory
- 3 A Model of Citric Acid Production in the Mold Aspergillus niger
- 4 Optimization Methods
- 5 Optimization of Biochemical Systems
- 6 Optimization of Citric Acid Production in Aspergillus niger
- 7 Maximization of Ethanol Production in Saccharomyces cerevisiae
- 8 Conclusions
- Author Index
- Subject Index
- Plate section
- References
Summary
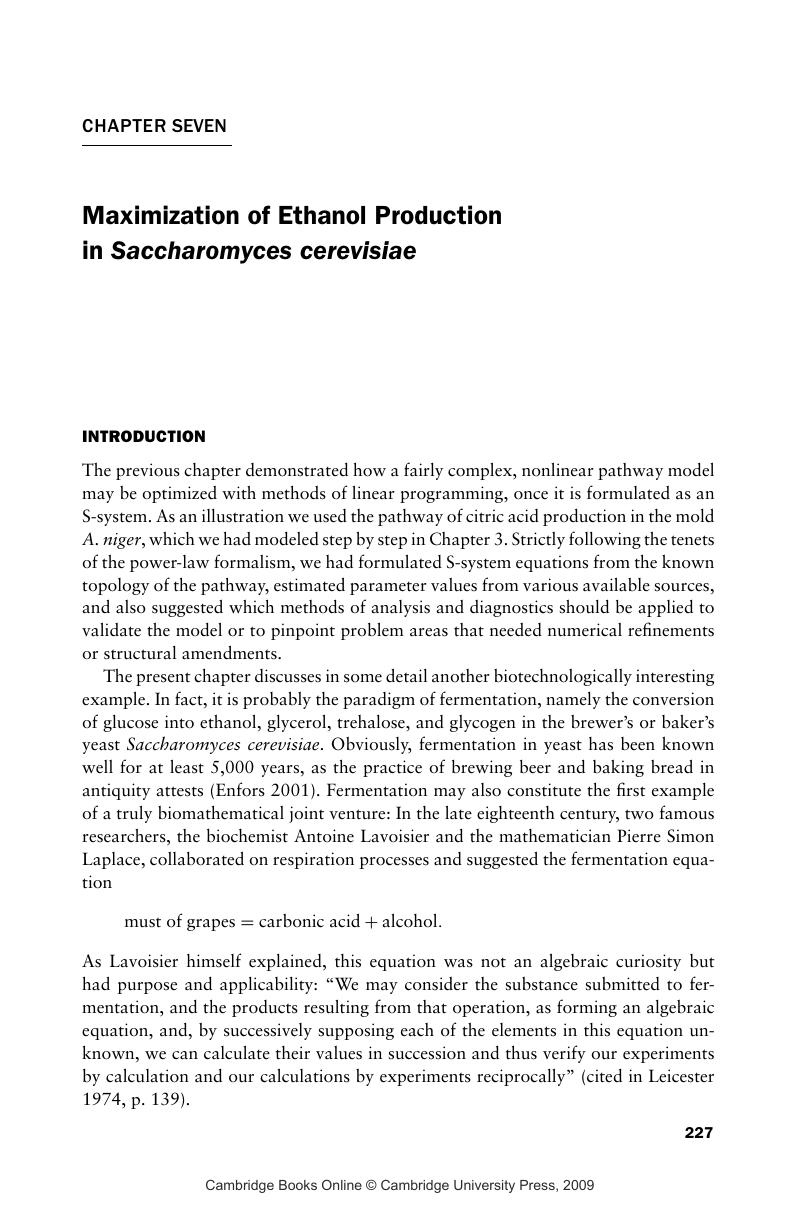
- Type
- Chapter
- Information
- Pathway Analysis and Optimization in Metabolic Engineering , pp. 227 - 272Publisher: Cambridge University PressPrint publication year: 2002