Book contents
- Essentials of Anesthesia for Infants and Neonates
- Essentials of Anesthesia for Infants and Neonates
- Copyright page
- Contents
- Contributors
- Preface
- Section 1 Newborn and Infant Physiology for Anesthetic Management
- 1 The Term Infant
- 2 The Preterm Infant
- 3 Origin of the NICU
- 4 Hemodynamics in the Infant
- 5 Measures of Carbon Dioxide
- 6 Glucose Control
- 7 Oxygen Management
- 8 Temperature Control
- 9 Neonatal Resuscitation
- 10 Congenital Abnormalities and Syndromes
- 11 Myopathies of the Newborn
- Section 2 Newborn and Infant Anesthesia
- Section 3 Specific Newborn and Infant Procedures
- Section 4 Pain Management and Other Newborn and Infant Anesthesia Concerns
- Index
- References
7 - Oxygen Management
Concerns About Both Hyperoxia and Hypoxia in Neonates During Critical Care and Intraoperatively
from Section 1 - Newborn and Infant Physiology for Anesthetic Management
Published online by Cambridge University Press: 09 February 2018
- Essentials of Anesthesia for Infants and Neonates
- Essentials of Anesthesia for Infants and Neonates
- Copyright page
- Contents
- Contributors
- Preface
- Section 1 Newborn and Infant Physiology for Anesthetic Management
- 1 The Term Infant
- 2 The Preterm Infant
- 3 Origin of the NICU
- 4 Hemodynamics in the Infant
- 5 Measures of Carbon Dioxide
- 6 Glucose Control
- 7 Oxygen Management
- 8 Temperature Control
- 9 Neonatal Resuscitation
- 10 Congenital Abnormalities and Syndromes
- 11 Myopathies of the Newborn
- Section 2 Newborn and Infant Anesthesia
- Section 3 Specific Newborn and Infant Procedures
- Section 4 Pain Management and Other Newborn and Infant Anesthesia Concerns
- Index
- References
Summary
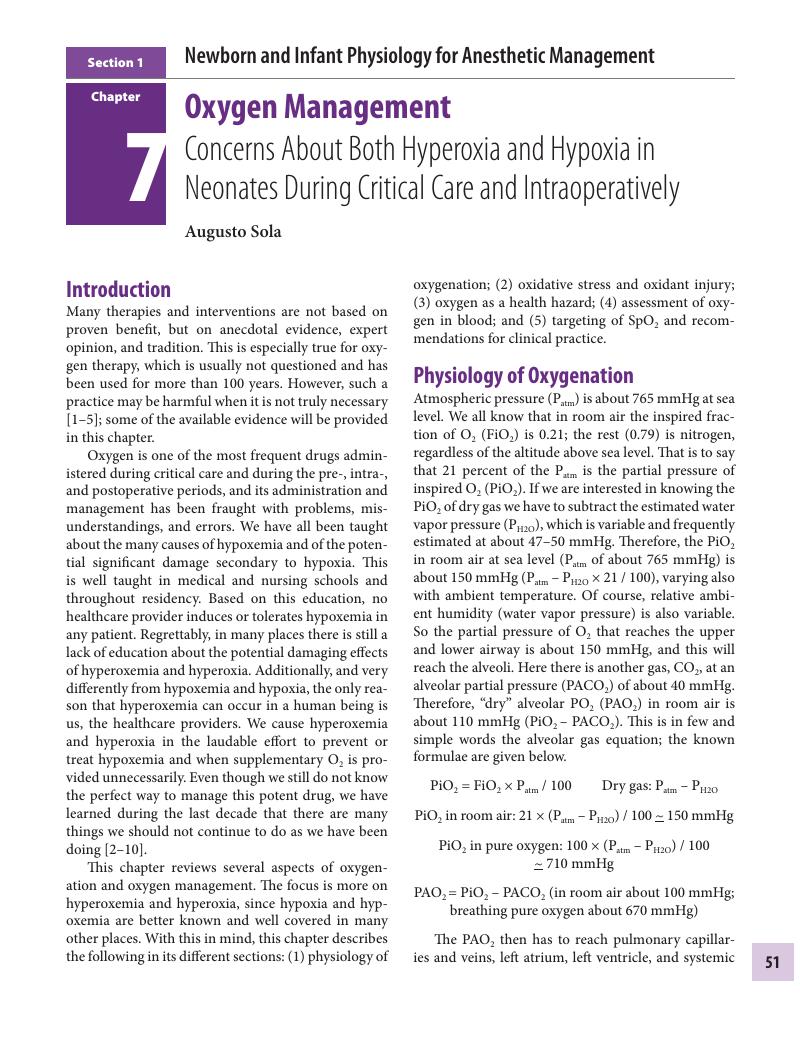
- Type
- Chapter
- Information
- Essentials of Anesthesia for Infants and Neonates , pp. 51 - 61Publisher: Cambridge University PressPrint publication year: 2018