Book contents
- Frontmatter
- Dedication
- Contents
- Foreword
- Preface
- 1 Nanoplasmonics
- 2 Thermodynamics of Metal Nanoparticles
- 3 Numerical Simulation Techniques
- 4 Thermal Microscopy Techniques
- 5 Thermal-Induced Processes
- 6 Applications
- Appendix A Dimensional Analysis
- Appendix B Thermodynamical Constants
- Appendix C Thermal Green's Function for a Three-Layer System
- Index
- References
5 - Thermal-Induced Processes
Published online by Cambridge University Press: 26 October 2017
- Frontmatter
- Dedication
- Contents
- Foreword
- Preface
- 1 Nanoplasmonics
- 2 Thermodynamics of Metal Nanoparticles
- 3 Numerical Simulation Techniques
- 4 Thermal Microscopy Techniques
- 5 Thermal-Induced Processes
- 6 Applications
- Appendix A Dimensional Analysis
- Appendix B Thermodynamical Constants
- Appendix C Thermal Green's Function for a Three-Layer System
- Index
- References
Summary
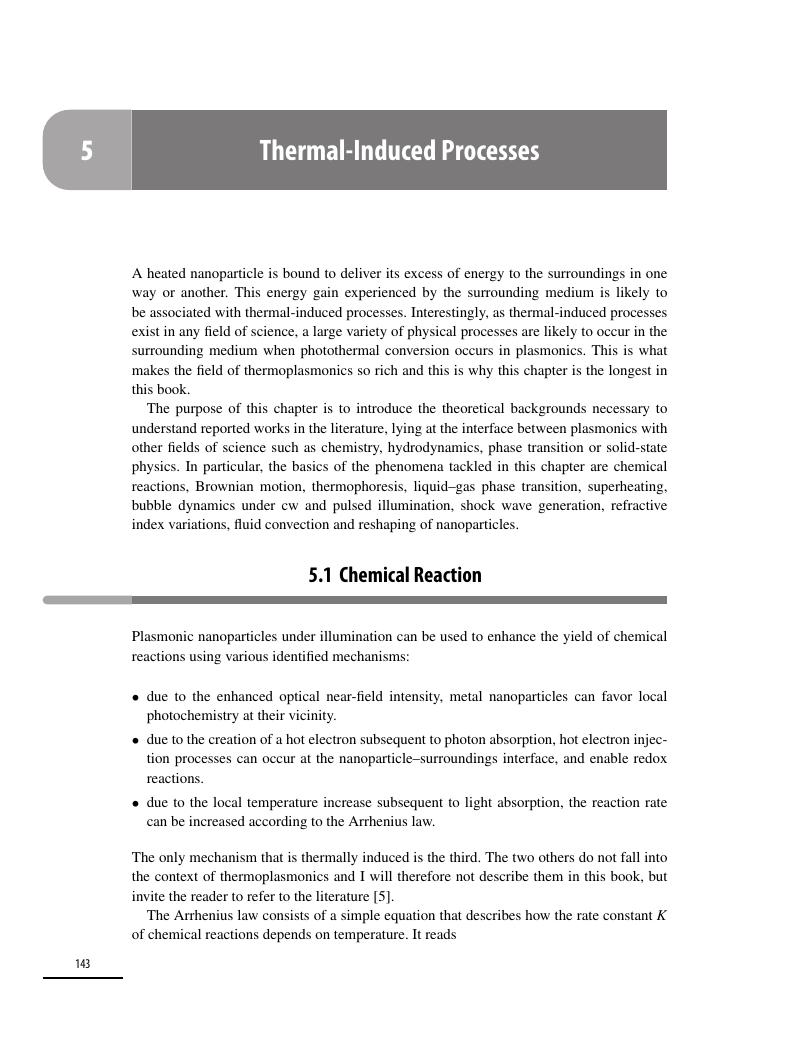
- Type
- Chapter
- Information
- ThermoplasmonicsHeating Metal Nanoparticles Using Light, pp. 143 - 222Publisher: Cambridge University PressPrint publication year: 2017