Introduction
Globally, bird populations are in decline (Şekercioğlu et al. Reference Şekercioğlu, Daily and Ehrlich2004, Butchart et al. Reference Butchart, Walpole, Collen, Van Strien, Scharlemann, Almond, Baillie, Bomhard, Brown, Bruno and Carpenter2010, BirdLife International 2018). Declines across Europe are most drastic in agricultural landscapes as a result of agricultural intensification and land-use changes (Chamberlain et al. Reference Chamberlain, Fuller, Bunce, Duckworth and Shrubb2000, Donald et al. Reference Donald, Green and Heath2001, Donald and Morris Reference Donald and Morris2005, Gregory et al. Reference Gregory, Van Strien, Vorisek, Meyling, Noble, Foppen and Gibbons2005, BfN 2017, Gregory et al. Reference Gregory, Skorpilova, Vorisek and Butler2019), an effect also documented for Germany (Sudfeldt et al. Reference Sudfeldt, Dröschmeister, Frederking, Gedeon, Gerlach, Grüneberg, Karthäuser, Langgemach, Schuster, Trautmann and Wahl2013, Wahl et al. Reference Wahl, Dröschmeister, Gerlach, Grüneberg, Langgemach, Trautmann and Sudfeldt2015).
The severe declines of farmland bird populations in Europe is well documented e.g. by a more than 50% reduction of the EU farmland bird indicator since 1980 (Gregory et al. Reference Gregory, Van Strien, Vorisek, Meyling, Noble, Foppen and Gibbons2005, Reference Gregory, Skorpilova, Vorisek and Butler2019, EBCC 2017). Negative population trends across Europe are mainly attributed to agricultural intensification as enforced by the European Common Agricultural Policy (CAP) (Donald et al. Reference Donald, Green and Heath2001, Reference Donald, Sanderson, Burfield and van Bommel2006, Assandri et al. Reference Assandri, Bogliani, Pedrini and Brambilla2019). This is also supported by studies from Eastern European countries, such as the Czech Republic, where strong declines have been observed especially after European Union (EU) accession where the CAP caused significant deterioration in farmland bird populations (Reif and Vermouzek Reference Reif and Vermouzek2018). Agricultural intensification comprises a variety of processes, acting simultaneously (Reif Reference Reif2013). Important specific aspects are, e.g. the shift from winter to spring cereals restricting winter food supply for seed eaters, the reduction of marginal habitats limiting breeding habitat for tree and shrub species, and increasing livestock numbers and mowing frequency on grasslands which destroys nesting sites (Newton Reference Newton2004, Reif Reference Reif2013). Also, ground-breeding farmland birds nesting on arable fields show the strongest declines among the species group in several studies (Heldbjerg et al. Reference Heldbjerg, Sunde and Fox2018, Jerrentrup et al. Reference Jerrentrup, Dauber, Strohbach, Mecke, Mitschke, Ludwig and Klimek2017) and generally species associated with arable land seem to suffer stronger declines than species associated with grassland and pasture (Reif and Hanzelka Reference Reif and Hanzelka2016, Heldbjerg et al. Reference Heldbjerg, Sunde and Fox2018). Additionally, changes in the availability of fallow land, largely determined by EU subsidised set-aside, have been identified to have strong effects on farmland bird populations (Henderson and Evans Reference Henderson, Evans, Aebischer, Evans, Grice and Vickery2000, Traba and Morales Reference Traba and Morales2019). A comprehensive assessment of factors influencing farmland bird populations in Germany at the national scale is however currently missing. For the German federal state of Lower Saxony, landscape homogenisation due to decreases in crop diversity and increasing cultivation of maize was identified to be negatively related to farmland bird population abundance (Jerrentrup et al. Reference Jerrentrup, Dauber, Strohbach, Mecke, Mitschke, Ludwig and Klimek2017).
Understanding the reasons for population change requires overcoming a sector-specific perspective, e.g. not focusing exclusively on effects related to agriculture. Despite the prominence and relevance of specific economic sectors or habitat-specific drivers of population change (e.g. agricultural intensification for farmland birds; Gamero et al. Reference Gamero, Brotons, Brunner, Foppen, Fornasari, Gregory, Herrando, Hořák, Jiguet, Kmecl, Lehikoinen, Lindström, Paquet, Reif, Sirkiä, Škorpilová, van Strien, Szép, Telenský, Teufelbauer, Trautmann, van Turnhout, Vermouzek, Vikstrøm and and Voříšek2017), regional variability in strength and composition of habitat-specific factors as well as large-scale factors, acting across habitats and at large spatial scales, shape population dynamics (Stephens et al. Reference Stephens, Mason, Green, Gregory, Sauer, Alison and Willis2016, Reif and Vermouzek Reference Reif and Vermouzek2018).
Regionally varying abiotic conditions such as geomorphology, elevation, slope, groundwater hydrology, soil conditions and weather strongly shape the state of natural as well as anthropogenically utilised areas (Chen et al. Reference Chen, Franklin and Lowe1996, Machado et al. Reference Machado, Bynum, Archer, Lascano, Wilson, Bordovsky, Segarra, Bronson, Nesmith and Xu2000). On farmland, abiotic conditions trigger the type of crop cultivated, field and pasture size and farming practice at regional level (Hendriks et al. Reference Hendriks, Stobbelaar and Van Mansvelt2000). Key factors causing population changes may therefore also differ regionally (Jerrentrup et al. Reference Jerrentrup, Dauber, Strohbach, Mecke, Mitschke, Ludwig and Klimek2017).
Overarching biological factors such as migration strategy (Sanderson et al. Reference Sanderson, Donald, Pain, Burfield and Van Bommel2006), anthropogenic pressures away from breeding sites like hunting on migration (McCulloch et al. Reference McCulloch, Tucker and Baillie1992), conditions at stopover sites (Yong and Moore Reference Yong and Moore1997) and on the wintering grounds (Morrison et al. Reference Morrison, Robinson, Clark, Risely and Gill2013) and climate change (Both et al. Reference Both, Bouwhuis, Lessells and Visser2006, Saino et al. Reference Saino, Ambrosini, Rubolini, Hardenberg, Provenzale, Hüppop, Hüppop, Lehikoinen, Lehikoinen, Rainio and Romano2011, Burns et al. Reference Burns, Eaton, Barlow, Beckmann, Brereton, Brooks, Brown, Al Fulaij, Gent, Henderson and Noble2016, Culp et al. Reference Culp, Cohen, Scarpignato, Thogmartin and Marra2017) are important drivers of abundance trends of individual species. As detailed above, policies acting at large spatial scales, like the CAP of the EU, additionally influence bird populations (e.g. Donald et al. Reference Donald, Pisano, Rayment and Pain2002, Pe’er et al. Reference Pe’er, Dicks, Visconti, Arlettaz, Baldi, Benton, Collins, Dieterich, Gregory, Hartig, Henle, Hobson, Kleijn, Neumann, Robijns, Schmidt, Shwartz, Sutherland, Turbe and Scott2014, Gamero et al. Reference Gamero, Brotons, Brunner, Foppen, Fornasari, Gregory, Herrando, Hořák, Jiguet, Kmecl, Lehikoinen, Lindström, Paquet, Reif, Sirkiä, Škorpilová, van Strien, Szép, Telenský, Teufelbauer, Trautmann, van Turnhout, Vermouzek, Vikstrøm and and Voříšek2017, Reif and Vermouzek Reference Reif and Vermouzek2018). Therefore, the analysis of drivers of population change requires the integration of regionally varying sectoral factors and overarching, large-scale pressures.
In this context our study examined whether the population trends of farmland bird species vary regionally across Germany and which factors cause long-term population change of individual species, in order to aggregate these results and derive conclusions for the overall group of farmland birds. The aim of our study is to identify and rank key drivers of population change according to their relative importance, to determine priorities for farmland bird conservation in Germany.
While similar studies have been carried out for several European countries in recent years (e.g. Chamberlain et al. Reference Chamberlain, Fuller, Bunce, Duckworth and Shrubb2000, Newton Reference Newton2004, Aunins and Priednieks Reference Aunins and Priednieks2008, Sanderson et al. Reference Sanderson, Kloch, Sachanowicz and Donald2009, Gil-Tena et al. Reference Gil-Tena, De Cáceres, Ernoult, Butet, Brotons and Burel2015, Heldbjerg et al. Reference Heldbjerg, Sunde and Fox2018, Reif and Vermouzek Reference Reif and Vermouzek2018, Traba and Morales Reference Traba and Morales2019) our case study closes a considerable spatial gap on the subject in Central Europe and combines the consideration of regionalised land-use change, weather variables and, where appropriate, further overarching factors, such as the conditions at staging or wintering grounds outside Europe to gain a comprehensive understanding of drivers of population change in common farmland birds in Germany.
Materials and methods
The study focuses on the period 1991–2013. Prior to analysing interrelationships between bird and environmental data, a comprehensive assessment of potential determining factors for bird populations was carried out to assure the inclusion of likely relevant factors for modelling, provided suitable statistical time series were available.
Considering the size of Germany, its geographic heterogeneity (coastal areas, lowland, uplands, Alps) and differing spatial structures of agricultural landscapes between East and West Germany as a consequence of the former division of Germany, all analyses were carried out subdivided by the regions north-west Germany (NW): representing the landscape type “Atlantic lowland” and West German land-use patterns; south-west Germany (SW) Figure S1: characterised especially by low mountain ranges and West German land-use patterns; and East-Germany (E): representing continental lowland and East German land-use patterns (Sudfeldt et al. Reference Sudfeldt, Dröschmeister, Wahl, Berlin, Gottschalk, Grüneberg, Mitschke and Trautmann2012) (Figure S1 in the online Supplementary Material). This enabled the assessment to account for the well-known geographical differences in population trends found by previous analyses (Rösler and Weins Reference Rösler and Weins1996, Schwarz and Flade Reference Schwarz and Flade2000, Flade and Schwarz Reference Flade and Schwarz2004, Flade Reference Flade2012) and to reflect potential regional variation in correlates of population change.
The use of species-specific models ensures that potentially relevant factors at species level are not overlooked as could be the case in a more general model at species group level, due to the considerable heterogeneity among the population trends of the farmland birds under study. This innovative species-specific and regionally stratified modelling approach moreover increased the robustness of the conclusions drawn, because consistent results are only generated if an effect is manifested in the different regions – despite their geographic heterogeneity and differing land-use histories.
Data
Species selection:
The species selection for the farmland birds investigated (Table 1; Table S1) was informed by three criteria. (1) The species’ breeding habitat and /or feeding habitat (depending on which is considered as a limiting factor for the population development of each species) during the breeding season, as defined for Germany by Wahl et al. (Reference Wahl, König, Grüneberg and Trautmann2014), had to represent one of the following types of agricultural land: arable fields and open land characterised by arable fields; farmed open land (indifferent) – a category used if no further differentiation of an agriculturally used open landscape is possible; grassland and open land characterised by grassland; or wasteland, ruderal and tall forb communities. (2) Reliable population trends from the German breeding bird monitoring schemes, primarily indicated by the respective standard error, had to be available at a regional scale for at least two of the three classified German regions, to make sure to focus on widely distributed species with a comprehensive data base. (3) Moreover, most relevant suspected population change determining factors for the selected species had to relate to agricultural practices. Those criteria were fulfilled for 16 species of 56 selected, comprising six national indicator species and five sub-national (federal) indicator species. Due to the indicator function of a considerable proportion of the selected species, covering various ecological niches of agricultural habitats, results are expected to be representative for the overall group of farmland birds (Achtziger et al. Reference Achtziger, Stickroth and Zieschank2004).
Table 1. Farmland bird species included in the analysis, their habitat and use as indicator species. For the overall species selection process see Table S1 in the online supplementary material.
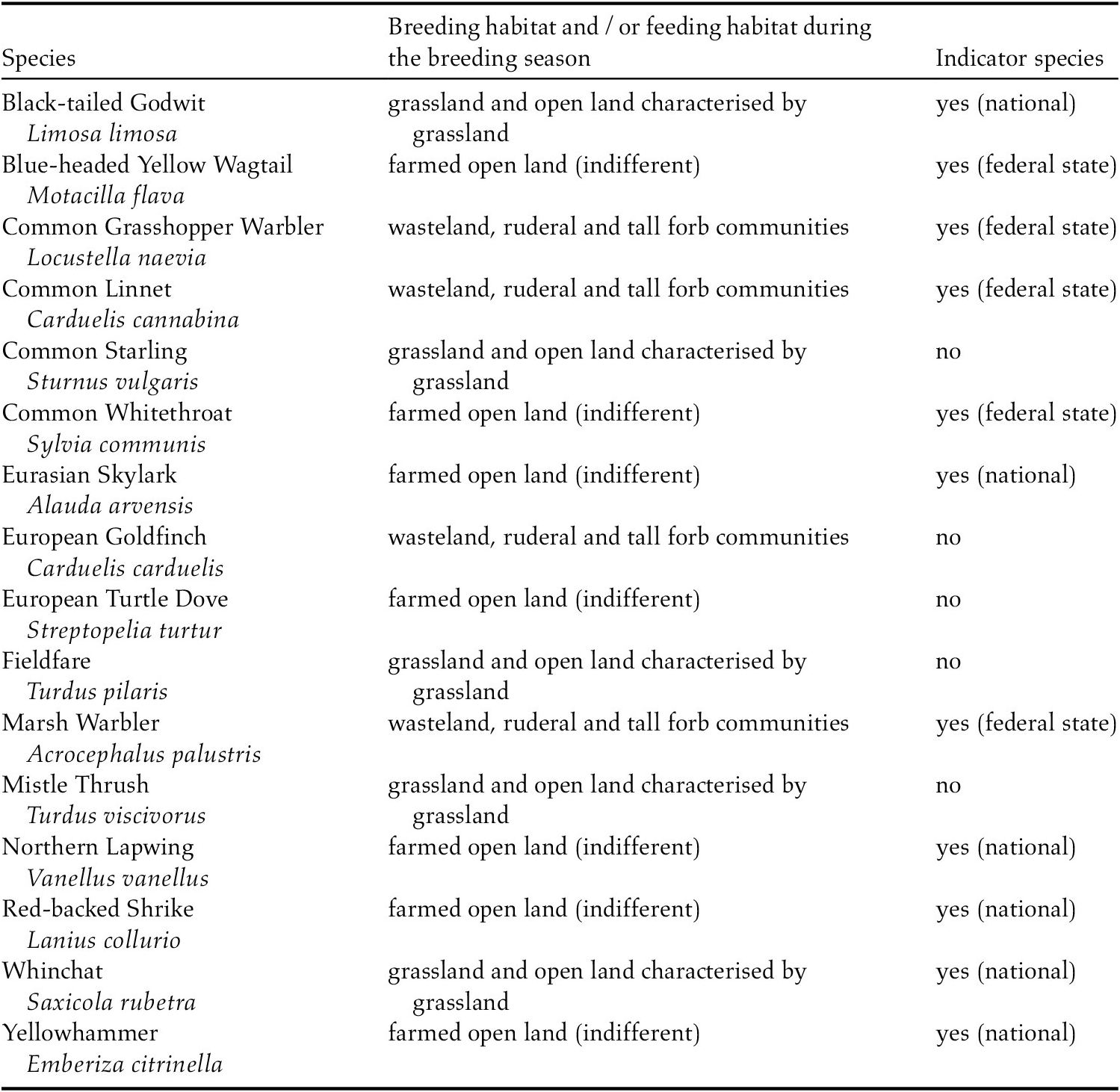
Bird data:
Information on population changes were available from the German breeding bird monitoring coordinated by the Federation of German Avifaunists (DDA). Annual population trend indices for the majority of species originate from the German Common Breeding Bird Survey (CBBS). The CBBS sampling plots are based on a stratified random sample, where sampling plots are randomly distributed across the different German regions analysed and adequately consider different habitat types (Mitschke et al. Reference Mitschke, Sudfeldt, Heidrich-Riske and Dröschmeister2005, Sudfeldt et al. Reference Sudfeldt, Dröschmeister, Wahl, Berlin, Gottschalk, Grüneberg, Mitschke and Trautmann2012). In 2013, the last year of our study period, 361 CBBS sampling plots were surveyed in the NW Germany region, covering the federal states North Rhine-Westphalia, Schleswig-Holstein, Bremen, Hamburg and Lower Saxony. For SW Germany, covering Rhineland-Palatinate, Baden-Württemberg, Bavaria, Hesse and Saarland (omitted because of data sparsity), 572 sampling plots were surveyed, and E Germany, comprising Brandenburg, Saxony, Saxony-Anhalt, Thuringia, Berlin and Mecklenburg-West Pomerania, contributed 579 sampling plots to the CBBS (Figure S1). Regionally stratified annual population trend indices for the investigation period 1991–2013 were calculated using the software TRIM (Pannekoek and van Strien Reference Pannekoek and van Strien2005) and indexed to the year 2006 (Figure 1).
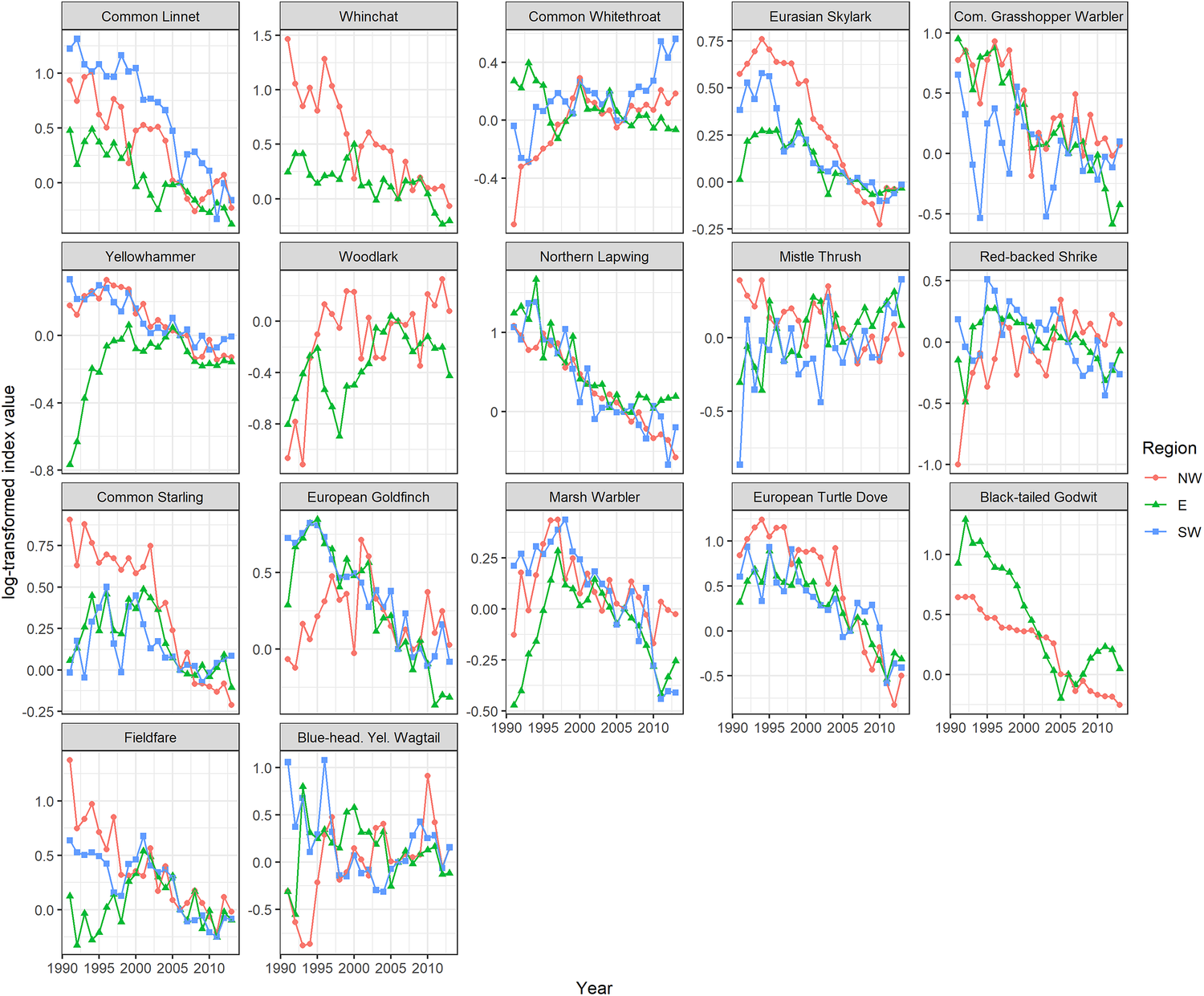
Figure 1. Population indices (log-transformed TRIM index values) for farmland birds 1991–2013, separately for three regions of Germany. NW = north-west Germany, E = East Germany, SW = south-west Germany.
A change in CBBS monitoring methodology, from point stop counts to line transect counts, during the investigation period is not expected to have a large impact on trends and their reliability as a close correlation of both methods could be found during 2005–2010, when both programmes were run in parallel. For one species, the Black-tailed Godwit Limosa limosa, indices originate from the German Rare Breeding Bird Survey (RBBS), based on annual population estimates at federal state level (Sudfeldt et al. Reference Sudfeldt, Dröschmeister, Wahl, Berlin, Gottschalk, Grüneberg, Mitschke and Trautmann2012). The regionally stratified population trend indices calculated illustrate considerable differences in the development of bird populations at regional scale, highlighting the necessity of a regionally stratified analysis (Figure 1).
Identifying potential pressures:
Initially, factors suspected to trigger population trends were identified by reviewing the national Birds Directive Report 2013, listing pressures and threats for German bird populations (Grüneberg et al. Reference Grüneberg, Dröschmeister, Fuchs, Frederking, Gerlach, Hauswirth, Karthäuser, Schuster, Sudfeldt, Trautmann and Wahl2017). Moreover, by comparing stratified population trends for NW, SW and E Germany, regional differences were detected (Figure 1) which supported the identification of potential drivers for population change.
This approach resulted in a preliminary list of factors potentially regulating population dynamics that should be tested in correlative analysis when described by suitable variables available at the required spatial and temporal scale. This selection process assured that only relevant causal factors underpinned by suitable data series for the overall group of farmland birds were included in the following correlative modelling framework to identify relevant drivers of population change.
Data on potential influencing biotic and abiotic variables:
Time series for potential population-determining factors used in our analysis were collated from various governmental, public as well as private sources and comprise land-use and weather variables. All variables listed in Table 2 were considered as potential drivers of population change for the 16 farmland bird species available for analysis. The only exception was the variable “SahelRainfall”, only tested for trans-Saharan migrants. Information on the development of the overall area used for the cultivation of various crops within the regions analysed, was compiled based on reports of statistical offices at national and federal state level or gathered from professional associations for particular crops, e.g. the German Maize Committee. To consider a change of the total agricultural area over time, the variables describing the spatial extent of different crop types (area in ha) were transformed to a percentage of the total agricultural area in the respective year and region before analysis.
Table 2. Variables included in models of farmland bird population change in Germany.
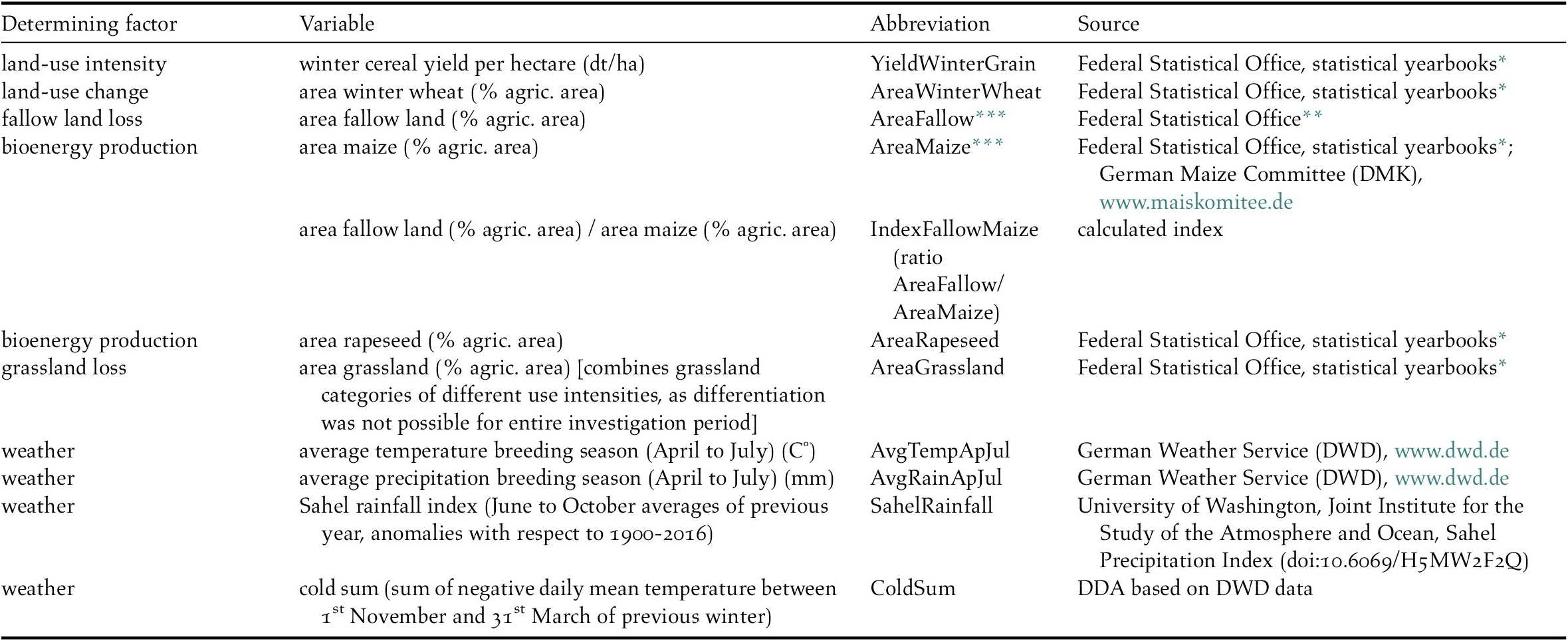
* Federal Statistical Office 1992-2014: Statistical Yearbooks for the Federal Republic of Germany. Federal Statistical Office, Wiesbaden.
** Federal Statistical Office 1992-2014: Fachserie 3, Reihe 3.1.2. Federal Statistical Office, Wiesbaden.
*** Variables AreaFallow and AreaMaize where combined in the IndexFallowMaize and therefore are not further mentioned in text and figures.
Weather variables, i.e. precipitation and temperature time series, known to be highly influential for birds (Lemoine and Bohning-Gaese Reference Lemoine and Bohning-Gaese2003), were obtained from the German Weather Service (DWD). For each of the three regions analysed, data from several (n = 8–12) weather stations distributed across the respective region were averaged to reflect regional differences in these weather variables and secure comparability with the representative bird trend indices calculated for the same spatial unit. Figure S2 illustrates the development of the land-use and weather variables over the investigation period and indicates the, in part, considerable regional differences.
The collation of suitable data was difficult for several potentially relevant determining factors mainly for two reasons: (1) The heterogeneity in terms of the spatial resolution of available data (national, federal, municipal level or point data) did often not allow us to rescale data to a common geographic unit and (2) time series varied in length, and often did not cover the entire investigation period. For inclusion into our analysis, annual data had to be available for the investigation period 1991–2013 at federal state level. This allowed us to merge data for the three regions investigated, each comprising several German federal states. While data for some relevant determining factors were not available to our project, suitable information for most relevant factors was gathered, enabling a relatively comprehensive assessment.
For the reasons described above, a site-level analysis, matching bird data of individual monitoring plots with environmental variables measured at the same spatial unit, was not an option as land-use data were not available at the required scale.
Statistical analysis
We fitted linear mixed-effects models (LMM) for each species to investigate which variables best explained the variation in population trend indices from 1991 to 2013. The yearly relative abundance of each species (TRIM population indices, log-transformed) in the three regions of Germany (NW, SW, E) was used as the response variable (Heldbjerg et al. Reference Heldbjerg, Sunde and Fox2018, Reif and Vermouzek Reference Reif and Vermouzek2018). Effect estimates from the LMMs can therefore be interpreted as percentage changes in the response variable. Assumptions of normality and homoscedasticity were assessed by plotting model residuals and accomplished by the data. To control for non-independence of the annual index values between the three regions, the LMMs were fitted with a random year intercept. To control for possible serial correlation, we tested the inclusion of an autoregressive term (Zuur et al. 2009), but for most species the model fit worsened as a consequence (mean increase in AIC by 0.2) and thus we did not include it further. The model residuals were also assessed with plots of the autocorrelation function but showed no clear sign of serial correlation. All explanatory variables (Table 2) were standardised by centring and scaling prior to analysis and are therefore directly comparable in their effect size (Schielzeth Reference Schielzeth2010). We also tested a time lag of one year, i.e. related the population index of year X+1 to the value of the explanatory variable in year X. The population responses to both weather and land-use variables differed little between time lags of null and one year (Figure S3 and S4). We also tested whether the population response differed significantly between the two time lags using univariate correlations (with function ‘paired.r’ from package ‘psych’), separately for each explanatory variable (all P-values for differences in correlation < 0.13). We therefore matched all variables from the same year (lag 0) for final analysis. All explanatory variables were tested for multicollinearity and we only included variables with a Pearson correlation coefficient of > 0.5 in the same model. The variables AreaGrassland, AreaFallow and AreaMaize showed correlations < 0.5 and these were accounted for by calculating a new index variable IndexFallowMaize (ratio of AreaFallow/AreaMaize) that was used in all models instead. After calculation of this index variable, the correlation with grassland was lower than the threshold of 0.5 (r = 0.43) and variance inflation factors > 3 in the fitted models assured that multicollinearity between predictors was successfully reduced (Zuur et al. Reference Zuur, Ieno and Elphick2010). The variable SahelRainfall was only included in models for species classified as long distance migrants with confirmed high dependence on the Sahel region according to Zwarts et al. (Reference Zwarts, Bijlsma, van der Kamp and Wymenga2012). Accordingly, this variable was used for Blue-headed Yellow Wagtail Motacilla flava, Common Whitethroat Sylvia communis, Marsh Warbler Acrocephalus palustris and Whinchat Saxicola rubetra, but not for Red-backed Shrike Lanius collurio. All predictor variables were fitted as additive fixed terms, a fixed effect for region (NW, SW, E) was also retained in all models. The limited amount of data available for each species did not allow testing for interaction terms in the models. All possible combinations of the predictor variables were tested as competing models; which model best explained variation in the response was assessed with the corrected Akaike Information criterion (AICc; Burnham and Anderson Reference Burnham and Anderson2002, Zuur et al. Reference Zuur, Ieno, Walker, Saveliev and Smith2009). Competing models within delta > 2 AICc of the best fitting model were selected for each species (Burnham and Anderson Reference Burnham and Anderson2002). The competing models selected for each species were averaged with the natural average method (Grueber et al. Reference Grueber, Nakagawa, Laws and Jamieson2011). This method was used to prevent zero-shrinkage of averaged estimates, as we also wanted to detect possible weak effects of predictor variables. The species effect size estimates and their uncertainty were then used to generate 1,000 parametric bootstrap samples each, which further provided a farmland species group estimate for the average effect of each variable with a measure of uncertainty. To measure model fit, the amount of variance explained by fixed and random effects in the LMMs was quantified with the marginal and conditional R2 method suggested by Nakagawa and Schielzeth (Reference Nakagawa and Schielzeth2013). To correct the marginal R2, the amount of variance attributable to the regional stratification in the population trend indices was estimated and subtracted from the variability attributable to other fixed effects in the models. All models were fitted in R v. 3.4.0 9 (R Core Team 2017) with the ‘lme4’ package v. 1.1-13 (Bates et al. Reference Bates, Maechler, Bolker and Walker2015). Model selection and averaging was done with the ‘MuMIn’ package v. 1.15.6 (Barton Reference Barton2016). For estimation of marginal and conditional R2 values the ‘r2glmm’ package v. 0.1.0 was used (Jaeger Reference Jaeger2016).
Results
The obtained species-specific models allow a detailed view of the combination of factors determining population changes and the relative strength of each variable (effect size) in the context of all variables selected by the best models (Figure 2). The analysis aimed at identifying a variety of factors determining population change, including also less prominent factors of smaller magnitude, to realistically investigate the likely interplay of a multitude of factors. The chosen modelling approach (averaging of best competing models selected for each species) ensured a comprehensive, multi-layered assessment for the group of farmland birds investigated.

Figure 2. Species-specific effect sizes averaged from competing LMMs with error bars showing a 95% CI. Estimates overlapping with 0 are coloured in grey. Note differing y-axis in subplots.
For the majority of farmland birds species-specific modelling results closely matched with those factors suspected to trigger population trends. For example, in case of Eurasian Skylark Alauda arvensis the availability of grassland showed a consistent positive effect on the species population index, also the availability of fallow land appears to have a positive effect on the species’ breeding abundance. At the same time increasing cultivation of rapeseed and winter wheat (mainly replacing spring-sown wheat) had a consistent negative impact on the species. Moreover, modelling results suggest that weather conditions slightly warmer and wetter than the average during the breeding season are favourable for Eurasian Skylark while high yields of winter grains, suggesting a fast and dense growth of grains in the respective year, and cold winters might negatively influence the population trend index. This matches well the findings of other studies investigating the impacts of farming practise and weather conditions on Eurasian Skylark in the UK (e.g. Dougall Reference Dougall1996, Donald and Morris Reference Donald and Morris2005). Modelling results for Northern Lapwing Vanellus vanellus showed a similar pattern. While a very similar set of variables, showing the same direction of effects, was selected to influence population indices, effect sizes between both species differed. While for Eurasian Skylark especially the availability of grassland was identified to show the most substantial effect on the species, for Northern Lapwing the cultivation of winter wheat was detected to have the strongest consistent effect on the population index.
At the same time, some modelling results for single variable-species interactions also contradicted the expectations on factors likely to determine population changes, e.g. the negative effect of grassland on the trend of Common Whitethroat (Figure 2) appears questionable, as the species is not closely associated with this habitat type, and may indicate that this variable masks another unknown effect that could not be parameterized. Nonetheless, such results were not excluded from further analysis. Precluding results contradicting original expectations, without traceable reasons for doing so, is generally not appropriate.
To be able to draw robust conclusions at group level and to weaken the effect of sporadic outliers with regard to single variables, effects were averaged across all species of the group of farmland birds. The results of this analysis at group level showed clear differences in the effect size estimates for the land-use and weather factors and thus allowed ranking them according to their relative importance (Figure 3).

Figure 3. Average effect sizes for the group of farmland birds. Filled blue squares represent averages from 1000 bootstrap replications where the 95% CI does not overlap with 0, for open squares the CI overlaps with 0. Light grey dots show species-specific effect size estimates used for bootstrapping. The sample size (n) is the number of species for which an effect of the respective variable was found.
The findings for the group of farmland birds show that in general, land-use had a more pronounced effect on population trends than weather factors. Five land-use and four weather factors were identified to be relevant in determining population changes across the group of farmland birds (Figure 3). Especially the index combining the highly correlated variables of area covered by fallow land and maize was identified to have a strong and consistent effect on farmland birds. The extent of grassland was identified as another important and consistent determinant of farmland bird populations. More grassland positively influences the abundance of breeding farmland birds, indicating that it provides more suitable breeding and/or feeding habitat than land cultivated with arable crops. Moreover, relatively strong negative effects of the increasing cultivation of rapeseed (consistent effect) and winter wheat (95% CI overlapped zero) were detected. The variable “YieldWinterGrain” (95% CI overlapped zero), thought of as a general measure of agricultural land-use intensity, was identified as relevant at the species level. However, the average estimate at group level only showed a marginal and highly variable effect, providing an unexpectedly weak indication of the negative effects of increasing land-use intensity on the group of farmland birds. Of the weather variables, average temperature during the breeding season was identified to have a consistent and comparably strong effect at group level (estimated 4% population change with 0.74°C deviation from the average). While generally high heterogeneity within the species group was detected, indicated by the scattering of data points in Figure 3 (light grey dots), variability was much smaller for weather variables than for land-use variables. In particular, the average rainfall during the breeding season is characterised by narrow confidence intervals (though the 95% CI slightly overlapped zero), providing a strong indication that some farmland birds profit from weather conditions slightly wetter than the average (estimated 2% population change with 40 mm deviation from the average). The cold sum shows a marginal and inconsistent negative effect, indicating that a slight majority of species may be negatively affected by cold winters. The comparably low explanatory power of the variable might partly relate to the fact that it was modelled for all species of the group of farmland birds irrespective of whether they are resident or migratory. This was done because winter conditions may well affect short and long-distance migrants, e.g. due to delayed start of the vegetation period after long winters influencing the conditions during the subsequent breeding season (Yalden and Pearce-Higgins Reference Yalden and Pearce-Higgins1997, Przybylo et al. Reference Przybylo, Sheldon and Merilä2000). Sahel rainfall, only considered for trans-Saharan migrants with dependence on the Sahel region, shows a marginal positive effect, indicating that species affected by this variable on average appear to profit from wetter conditions in the Sahel region, which corresponds to various other studies (e.g. Szep Reference Szep1995, Boano et al. Reference Boano, Bonardi and Silvano2004, Zwarts et al. Reference Zwarts, Bijlsma, van der Kamp and Wymenga2012).
Model fit differed considerably across species, ranging from 70% explanatory power in case of the Northern Lapwing, to 7% in case of the Red-backed Shrike (Table 3). Average explanatory power (corrected marginal R2) across species was 42%, demonstrating that the variables are able to explain a considerable percentage of the variation within the trends of the farmland birds investigated.
Table 3. Model fit from averaged candidate models relating bird trends to land-use and weather variables (LMMs). The marginal R values shown in the table are corrected by excluding the variance attributable to the regional stratification in the bird trend data.

Discussion
The results obtained confirm and differentiate effects triggering population changes of farmland birds in Germany at national scale, bringing together environmental variables and bird monitoring data. Our analysis largely conforms to hypotheses concerning the conservation issues for farmland birds arising from modern agriculture, regularly formulated based on regional or local studies and incorporating broad experience with farmland bird conservation from ornithological experts (e.g. DDA and DO-G 2011). The methodology applied enabled the simultaneous consideration of various factors with the potential to drive population changes in farmland birds and - by comparing effect sizes among the selected variables - allowed disentangling the relative importance of individual drivers of population change.
Our approach allowed us to identify the transformation of fallow land, mainly into intensively farmed maize fields, as a highly relevant process for understanding and explaining the negative population trends of many farmland bird species in Germany during the period investigated (1991–2013). While more fallow land (and less maize) was related to population increases, the conversion of fallow land to maize, a process that was observed in Germany especially after 2007 when the EU fallow land subsidies were abolished (Osterburg et al. Reference Osterburg, Nitsch, Laggner and Roggendorf2009, Wahl et al. Reference Wahl, Dröschmeister, Gerlach, Grüneberg, Langgemach, Trautmann and Sudfeldt2015) and generally due to the strong increase of maize cultivation as energy crop (Nitsch et al. Reference Nitsch, Osterburg, Roggendorf and Laggner2012), represents the most pronounced and consistent driver of population change identified. Negative effects of increasing maize cultivation on farmland bird diversity in Germany are also identified by Sauerbrei et al. (Reference Sauerbrei, Ekschmitt, Wolters and Gottschalk2014). The loss of fallow land is likely to affect farmland bird species via the loss of habitat for breeding birds, as well as the loss of valuable food sources for granivorous sedentary birds and partial migrants during winter (Henderson and Evans Reference Henderson, Evans, Aebischer, Evans, Grice and Vickery2000, Sudfeldt et al. Reference Sudfeldt, Dröschmeister, Flade, Grüneberg, Mitschke, Schwarz and Wahl2009, Traba and Morales Reference Traba and Morales2019). Moreover, insect abundance is likely to be much higher on fallow land than on cultivated field blocks (Traba and Morales Reference Traba and Morales2019), e.g. because it is not treated with insecticides and accordingly the loss of fallow land may also reduce food availability for insectivorous species. Studies from other European countries also identified fallow land as one of the most important habitats in agricultural landscapes, particularly for farmland birds (Traba and Morales Reference Traba and Morales2019).
Another variable, “YieldWinterGrain”, showed an unexpectedly weak effect at group level, which was surprising considering its relevance indicated by similar studies as a proxy variable for increasing land-use intensity. While cereal yield per hectare is successfully used in studies at larger spatial scale (e.g. Gamero et al. Reference Gamero, Brotons, Brunner, Foppen, Fornasari, Gregory, Herrando, Hořák, Jiguet, Kmecl, Lehikoinen, Lindström, Paquet, Reif, Sirkiä, Škorpilová, van Strien, Szép, Telenský, Teufelbauer, Trautmann, van Turnhout, Vermouzek, Vikstrøm and and Voříšek2017) to measure land-use intensity and explained over 30% of the variation of farmland bird population trends at European scale (Donald et al. Reference Donald, Green and Heath2001), this variable appears not ideal for a German context. We assume this is due to the fact that the yield per hectare was already at a very high level in Germany during the investigation period and two- to threefold higher than in most southern and eastern European countries (http://www.fao.org/faostat/en/#data/QC). Negative effects of agricultural intensification therefore, to a considerable degree, are likely already reflected within the farmland bird population trends analysed. While yield per hectare still increases in Germany, the most profound impacts of intensification, as measured via cereal yield per hectare, most likely have been realised ahead of the investigation period. Today especially large-scale changes of the crop types cultivated and the transformation of extensive land-use practices (e.g. fallow land) seem to drive farmland bird population trends.
An aspect requiring discussion when interpreting the results is data availability. Evidently, suitable data for a few important factors determining population change were not available for the modelling process. Factors such as pesticide usage (Newton Reference Newton2004) and associated impact on food supply for birds (Hallmann et al. Reference Hallmann, Foppen, van Turnhout, de Kroon and Jongejans2014, BfN 2017), loss of landscape heterogeneity (Tscharntke et al. Reference Tscharntke, Klein, Kruess, Steffan-Dewenter and Thies2005, Verhulst et al. Reference Verhulst, Baldi and Kleijn2004), predation by mammals (Benton et al. Reference Benton, Vickery and Wilson2003, Evans Reference Evans2004) and modification of ground water level by drainage (Milsom et al. Reference Milsom, Langton, Parkin, Peel, Bishop, Hart and Moore2000, Kleijn Reference Kleijn and van Zuijlen2004) have been shown to affect bird populations, but despite comprehensive review and testing potential proxy variables, no suitable parameters describing these factors at the required temporal and spatial resolution were available for Germany. For pesticides, e.g. only data on annual domestic sales of active ingredients at national scale were available. But domestic sales do not necessarily correspond to the actual annual usage of pesticides in agriculture and usage strongly depends on the local weather conditions. Also, pesticide application differs regionally and the fact that data were only available on a national scale prevented the stratification into regions as required by the modelling framework. Availability of meaningful variables describing overall pesticide usage, or better separating between insecticides, herbicides and fungicides, are likely to further improve the modelling results. Especially in light of the obligation of farmers to collect all those measures at field and/or farm level (BVL 2014), it is unsatisfactory that such data are not available for the assessments presented here and similar studies like e.g. Jerrentrup et al. (Reference Jerrentrup, Dauber, Strohbach, Mecke, Mitschke, Ludwig and Klimek2017).
Also, little data on landscape structure were available, i.e. hedges, tree rows and other linear structures enriching agricultural landscapes. Modelling results need to be interpreted in this context, to better understand and assess e.g. the comparably low explanatory power of the Red-backed-Shrike model (7%), or inconsistencies of the Common Whitethroat model, both associated with hedgerows. Despite achieving high explanatory power, the Common Linnet Carduelis cannabina model (54%), representing the third hedge breeder in the group of farmland birds analysed, can be expected to further improve if a suitable variable for landscape elements was included into the model. Fortunately, a suitable dataset describing this factor, the national monitoring of high nature-value farmland (HNV) (Benzler Reference Benzler, Oppermann, Beaufoy and Jones2012), collecting data on the same sample plots as the CBBS, started in 2009 and therefore will provide suitable time series for inclusion in similar studies in future. Initial tests using HNV data indicated consistent relationships between hedge breeders and the extent and length of structural elements like field shrubs and hedges, highlighting the relevance of this factor.
Another aspect requiring attention is the parameterization of habitat quality for specific land-use types. In case of grassland areas, for example, it was not possible to differentiate between use intensities, because the classification of grassland categories measured annually by the Federal Statistical Office changed during the investigation period of this study (Federal Statistical Office 2009, 2011). Accordingly, we had to use a general grassland category as predictor in our models. However, recent evidence shows that while the quantitative loss of grassland slowed down in recent years, the qualitative deterioration of grasslands in Germany proceeds (Benzler et al. Reference Benzler, Fuchs and Huenig2015) indicated e.g. by a loss of 9% high nature value grassland in Germany during the period 2009–2015 (BfN 2017). Against the background that our study identified grassland as an important factor determining population change in farmland birds in Germany, a more differentiated review considering varying use intensities would be beneficial (e.g. Strebel et al. Reference Strebel, Jacot, Horch and Spaar2015).
In terms of further improving our ability to investigate and identify, but also monitor drivers of farmland bird population changes in Germany, the annual monitoring of a representative set of sample plots should therefore not be restricted to mapping birds. Ideally, bird surveys would be supplemented with regular monitoring of potentially important drivers of population change. This could help to measure spatially explicit effects which are acting at plot level rather than at a larger spatial scale (such as weather or climatic effects). Collecting data at plot level would be useful for variables relating to land-use change (habitat type and extent), habitat quality (e.g. diversity of plant species) and land-use intensity (e.g. input of fertilizers or cultivation cycles). In particular, spatial data regarding the type and intensity of cultivation at the level of monitoring plots would be useful to relate them to bird population trend data based on the same plots. However, measures of landscape connectivity (isolation and fragmentation), disturbance (human pressure) or diversity (especially regarding habitat features such as hedges etc.) should be supplemented at landscape scale.
Apart from factors shaping the extent and quality of agricultural habitats, overarching factors have the potential to influence bird species irrespective of their ecological niche, habitat association and season. Therefore, weather conditions during the breeding season (average temperature and precipitation) as well as non-breeding season (cold sum) were included into our analysis. By including the Sahel rainfall index as an explanatory variable for long-distance migrants, another large-scale factor was included into the analysis and selected in most species-specific models for trans-Sahara migrants. While climate change, clearly representing another important general factor, was not included by a specific variable into the analysis, this aspect was partly considered via the weather variables included in the analysis and results for average temperature and precipitation variables may be interpreted in the context of climate change. Suitable data to parameterise further overarching factors such as hunting pressure outside Germany (Hirschfeld and Attard 2018) were unfortunately not available or not suitable for inclusion into the model.
To illustrate how changes in agricultural management could influence populations of farmland birds in Germany and to point out the implications for farmland bird conservation, we extrapolated the average effect size estimates for the group of farmland birds and the land-use data for the factors identified as most relevant. This scenario was based on the most recent data in our model (year 2013) and built around a commonly discussed threshold of 10% agricultural land set aside from production to benefit farmland biodiversity (Sudfeldt et al. Reference Sudfeldt, Dröschmeister, Flade, Grüneberg, Mitschke, Schwarz and Wahl2009, DO-G Fachgruppe Agrarvögel 2012, Oppermann et al. Reference Oppermann, Kasperczyk, Matzdorf, Reuter, Meyer, Luick, Stein, Ameskamp, Gelhausen and Bleil2013, Reference Oppermann, Fried, Lepp, Lepp and Lakner2016). We modelled the effects of the different variables as additive, which surely represents a simplification. Nevertheless, based on our results, increasing the area of fallow land from 1.6% in 2013 to 10% of the total agricultural area in Germany (increase of 1,465,000 ha) would on average mean a 60% increase of the populations of farmland birds considered in our analysis. It has to be noted, that due to our model parameterization with an index of area fallow and area maize, this effect is based on a simultaneous decrease of the same area planted with maize. Additionally, if a) the nationwide percentage of grassland is increased from about 27% to 30% (by 390,000 ha; retaining ratios of high and low quality types) and b) about 215,000 ha sown with rapeseed are restored to a representative mix of prior use, these measures would on average increase the farmland bird populations by another 17% and 14% respectively. Thus, according to our scenario based on the model results, a far-reaching change in agricultural management would lead to an increase of the farmland bird populations in Germany of up to 90% compared to the year 2013 - by cutting down on bioenergy production, setting aside land for conservation and by preservation and restoration of cultivated grasslands. This scenario is based on the simplifying assumption that effects are additive and neither amplify nor complement each other. In order to estimate realistic joint effects, more sophisticated model projections would be needed in a predictive analytical framework (e.g. Butler et al. Reference Butler, Boccaccio, Gregory, Vorisek and Norris2010), but our scenario helps to gauge the magnitude such changes would produce.
The discussed changes in agriculture might be supported by consequently linking agricultural subsidies to improvements of biodiversity and at the same time preventing subsidies leading to the deterioration of bird diversity and abundance as recorded in the past decades. To gain more support by agriculture for biodiversity protection, a suite of financial incentives is needed to set up measures proven to be biodiversity friendly (Pe’er et al. Reference Pe’er, Dicks, Visconti, Arlettaz, Baldi, Benton, Collins, Dieterich, Gregory, Hartig, Henle, Hobson, Kleijn, Neumann, Robijns, Schmidt, Shwartz, Sutherland, Turbe and Scott2014).
Conclusion
This study for the first time quantitatively underpins the relevance of several factors associated with farmland bird population changes in Germany that formerly were discussed solely based on univariate correlations (e.g. Flade Reference Flade2012). The results highlight the major importance of fallow land and grassland areas in agricultural landscapes in supporting farmland bird species and indicate that especially the increasing cultivation of energy crops like maize and rapeseed appear to have negatively influenced the group of farmland bird species investigated during the period 1991–2013. The results obtained also demonstrate that despite the consistent influence of weather conditions during the breeding season, land-use changes clearly appear to have a stronger impact on bird populations - matching the results of similar studies from other European countries (Eglington and Pearce-Higgins Reference Eglington and Pearce-Higgins2012, Bowler et al. Reference Bowler, Heldbjerg, Fox, O’Hara and Böhning‐Gaese2018).
Translating the results obtained into recommendations for conservation measures to support farmland birds is facilitated by ranking the factors analysed according to their relative importance. Considering the limited funds available for nature conservation measures in agricultural landscapes, such prioritisation might support the efficient use of these limited financial resources to benefit farmland birds. According to the findings of our study, the availability of extensively used areas (e.g. fallow land) appears to be the key for safeguarding and supporting the group of farmland birds. The need for practical measures to increase the extent and ecological quality of fallow land and grassland have been regularly formulated by various statutory (e.g. BfN 2014, 2017) and non-statutory nature conservation bodies in Germany (e.g. NABU 2009, Sudfeldt et al. Reference Sudfeldt, Dröschmeister, Flade, Grüneberg, Mitschke, Schwarz and Wahl2009, DDA and DO-G 2011, Wahl et al. Reference Wahl, Dröschmeister, Gerlach, Grüneberg, Langgemach, Trautmann and Sudfeldt2015) and at European scale (e.g. Pe’er et al. Reference Pe’er, Dicks, Visconti, Arlettaz, Baldi, Benton, Collins, Dieterich, Gregory, Hartig, Henle, Hobson, Kleijn, Neumann, Robijns, Schmidt, Shwartz, Sutherland, Turbe and Scott2014), and our results support these recommendations. The implementation of such measures will largely depend on successful adjustments during the current CAP funding period and, even more, a comprehensive reform and reorientation of the CAP to effectively facilitate biodiversity conservation ahead of the next funding period starting in 2020 (BfN 2017). In addition to environmentally sound regulations for farming practices, this should include an attempt to engage more strongly with municipalities, regional and local authorities to foster the protection of extensively used, semi-natural elements within agricultural landscapes. For example measures such as avoiding the regular cutting of vegetation between (dirt) roads and field margins or drainage diggings regularly bordering field blocks or roads, could potentially be enforced via legal guidelines to secure those marginal structures, providing, at smaller scale, similar ecological benefits as extensive grassland or fallow land areas.
Supplementary Material
To view supplementary material for this article, please visit https://doi.org/10.1017/S0959270919000480
Acknowledgements
The work presented was supported by the Federal Agency for Nature Conservation (BfN) with funds provided by the German Federal Ministry for the Environment, Nature Conservation, Building and Nuclear Safety (BMUB) in the framework of the Research and Development project “Analyzing causes for population changes in indicator species and energy transition” (FKZ: 3514 82 1000). The results presented do not necessarily also represent the position of the BfN or the BMUB. Moreover, we would like to thank all members of the project advisory group. Also, we are thankful for the constructive comments from two anonymous reviewers that helped to improve the quality of the manuscript.