Introduction
Antimicrobial resistance (AMR) is a problem of increasing public health concern worldwide, as antibiotics are among the most prescribed classes of drugs in human medicine. However, they are also largely used for therapy of a wide range of infections in animals and as prophylactic agents to prevent the development of infections in herds, as well as growth promoters in healthy livestock. Such extensive usage has been widely implicated in the selection of bacterial resistance [1]. To combat this effectively, it is recognised that a multidisciplinary approach including human and animal health, food production and environmental factors is necessary, in alignment with the World Health Organization One Health concepts [2].
The indiscriminate use of antibiotics in food-producing animals can lead to bacterial resistance, which can be transmitted to humans through three distinct ways: (i) by direct contact between humans and animals; (ii) through preparation and consumption of contaminated food, and (iii) indirectly, due to the excretion of resistant bacteria and unmetabolised antibiotics by animals, thereby causing additional selective pressure in the environment [Reference O'Neil3]. Thus, it is very important to include the food production chain in surveillance programmes on AMR, to provide information that enables the adoption of measures of prevention and control of AMR in this sector.
According to the WHO recommendations, integrated surveillance programmes should encompass foodborne pathogens or sentinel microorganisms in human clinical samples, retail foods and healthy food production animals, in this order of priority. It is noteworthy that food of animal origin represents an important route of human exposure to antimicrobial-resistant pathogens. Salmonella is usually one of the bacterial groups considered to be of high priority in surveillance programmes in the food chain [4].
A recent WHO report has highlighted that the development and implementation of national action plans on AMR is occurring in different stages among countries [5]. As such plans have recently been developed in Brazil [6, 7], the objective of this study was to provide baseline information to support the construction of a national integrated surveillance programme on AMR in foodborne pathogens in the country. Considering that Brazil is the second largest producer of poultry meat in the world (13 245 million tons) and exports approximately 32% of its production to the international market [8], we have investigated the serotype distribution and AMR of Salmonella isolates recovered from this production chain in Brazil, in two different years, 2014 and 2017, to identify possible trends or changes over this period.
Materials and methods
Isolates selection
A total of 170 Salmonella isolates from each assessed year (2014 and 2017) were randomly selected in a systematic model from the Brazilian Ministry of Agriculture, Livestock and Food Supply (MAPA) collection. The number of isolates examined was based on the Decision 2013/652/EU from the European Commission [9], which proposed that a sample size of 170 isolates per year for antimicrobial susceptibility testing was required for countries producing more than 100 000 tons of slaughtered poultry meat per year. However, due to problems in the storage conditions of the collection, we were only able to recover and test 146 and 163 Salmonella isolates from the years of 2014 and 2017, respectively.
Salmonella identification and serotyping
All isolates were obtained from poultry carcasses by the MAPA's laboratory network according to ISO 6579 procedures [10, 11]. Serotypes were determined by automated ribotyping, using RiboPrinter™System (DuPont Qualicon), according to the manufacturer's instructions [Reference Bailey12].
Antimicrobial susceptibility testing
Minimal inhibitory concentrations (MIC) of 13 antimicrobials were determined for the Salmonella spp. isolates by broth microdilution, according to ISO 20776-1:2006 [13]. The tested antibiotics are listed in Table 1, as well as the range of concentrations and the EUCAST clinical breakpoints [14]. For those antibiotics without defined breakpoints for Salmonella, the results were evaluated according to the European Food Safety Authority (EFSA) recommendations [15]. Isolates were characterised as multidrug-resistant (MDR) if resistant to three or more antimicrobial classes [Reference Magiorakos16]. Escherichia coli ATCC 25922, Staphylococcus aureus ATCC 29213 and Pseudomonas aeruginosa ATCC 27853 were used as quality control strains.
Table 1. Antimicrobials range of concentration and interpretative criteria used for testing Salmonella isolates from poultry meat

a EUCAST clinical breakpoint v 9.0 – European Committee on Antimicrobial Susceptibility Testing resistance breakpoint.
b No current EUCAST clinical breakpoint available. Complementary interpretative thresholds adopted as suggested by EFSA (2019).
c Trimethoprim/sulfamethoxazole in the ratio 1:19. Breakpoints are expressed as the trimethoprim concentration.
Statistical analysis
Statistical analysis was performed using WinPepi version 11.65. For all proportion estimates, exact binomial 95% confidence intervals (CIs) were calculated. Pearson's χ 2 was used to compare resistance rates between the periods evaluated (P < 0.05 was considered statistically significant).
Results
Geographical distribution of isolates
In the first evaluated period (January to November 2014), the 146 isolates were recovered from 58 different slaughterhouses under federal inspection, in 56 cities and 11 states. In the second period (March to December 2017), the 163 isolates were from 82 slaughterhouses, in 78 cities and 12 states (Fig. 1).
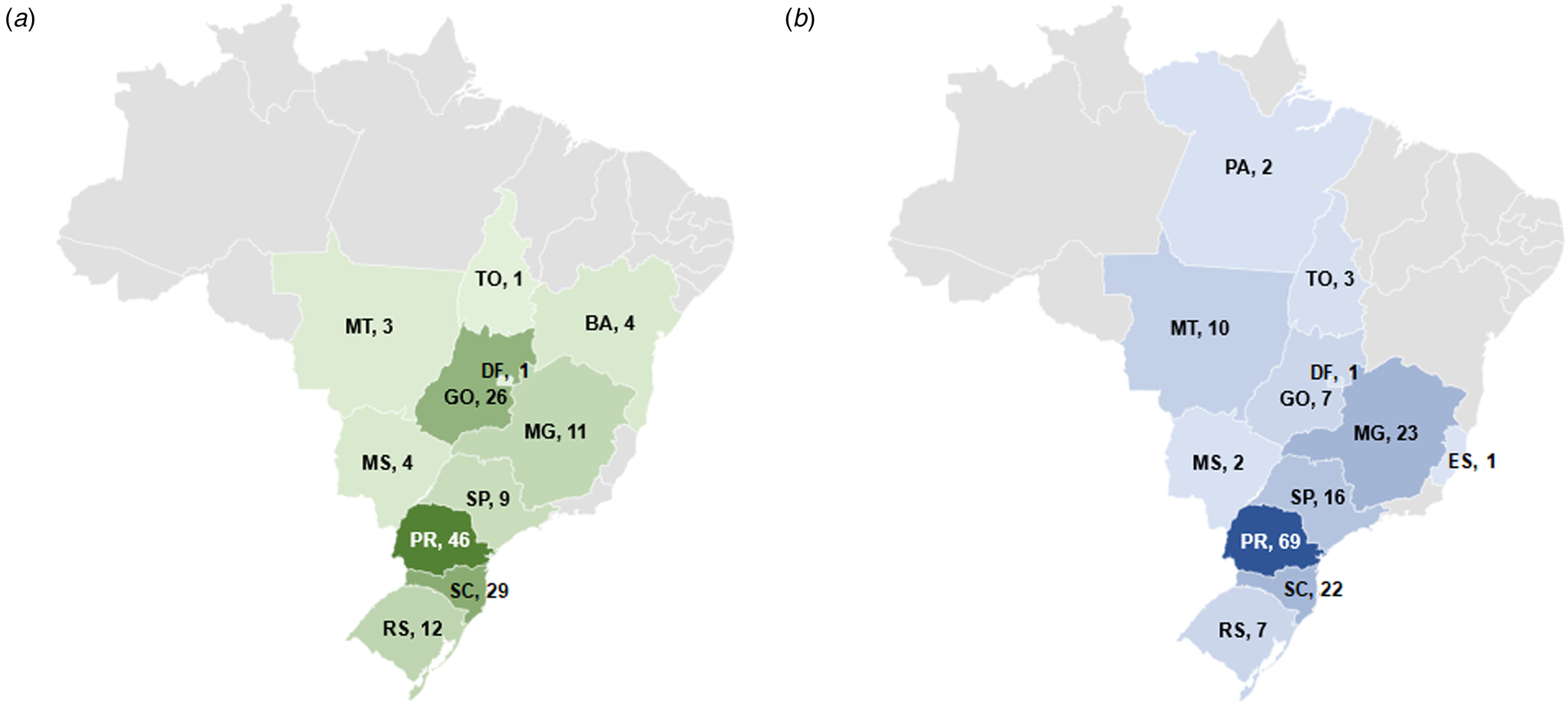
Fig. 1. Number of Salmonella isolates from 2014 and 2017 selected per state in Brazil. (a) 2014; (b) 2017. BA, Bahia; DF, Distrito Federal; ES, Espírito Santo; GO, Goiás; MG, Minas Gerais; MS, Mato Grosso do Sul; MT, Mato Grosso; PA, Pará; PR, Paraná; RS, Rio Grande do Sul; SC, Santa Catarina; SP, São Paulo; TO, Tocantins.
Serotype distribution
In total, 29 different serotypes were identified among the 146 Salmonella isolates from 2014 (Table S1). The most frequent were Salmonella ser. Heidelberg (55, 37.7%), Salmonella ser. Minnesota (17, 11.6%), Salmonella ser. Schwarzengrund (10, 6.8%), Salmonella ser. Infantis (9, 6.2%) and Salmonella ser. Saintpaul (6, 4.1%). The isolates from 2017 fell into 18 serotypes (Table S1), with Salmonella ser. Heidelberg (89, 54.6%), Salmonella ser. Minnesota (38, 23.3%) and Salmonella ser. Saintpaul (8, 4.9%) being the most frequent. The evaluation of the geographical distribution of the two most prevalent serotypes indicated that Salmonella ser. Heidelberg was identified in samples from four states in 2014, and in eight states in 2017. Likewise, Salmonella ser. Minnesota was recovered in six and nine states in 2014 and 2017, respectively (Fig. 2).
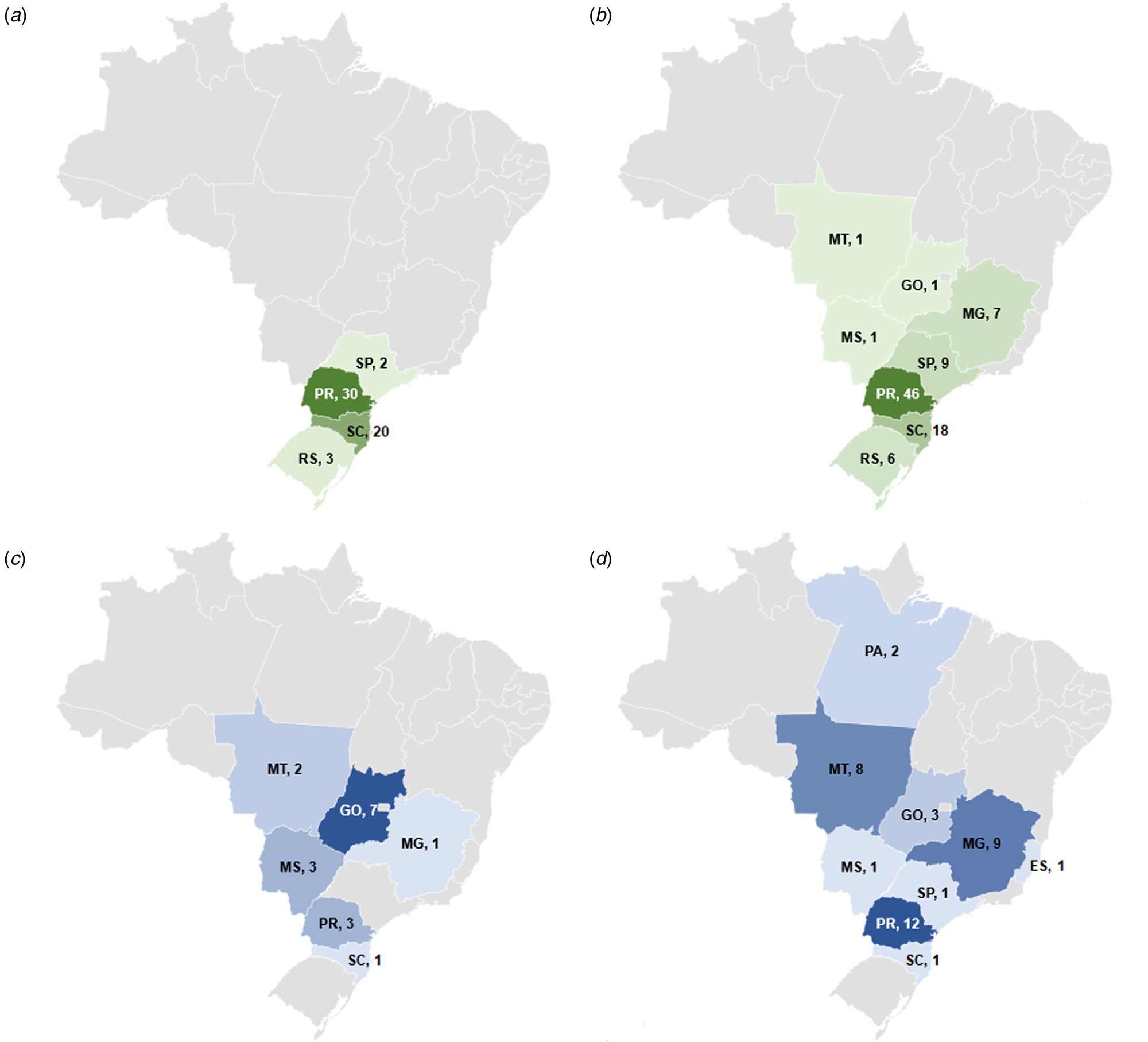
Fig. 2. Number of isolates and geographical distribution of the most prevalent serotypes of Salmonella from poultry meat in Brazil in 2014 and 2017. (a) Geographic distribution of Salmonella ser. Heidelberg in 2014; (b) geographic distribution of Salmonella ser. Heidelberg in 2017; (c) geographic distribution of Salmonella ser. Minnesota in 2014; (d) geographic distribution of Salmonella ser. Minnesota in 2017. ES, Espírito Santo; GO, Goiás; MG, Minas Gerais; MS, Mato Grosso do Sul; MT, Mato Grosso; PA, Pará; PR, Paraná; RS, Rio Grande do Sul; SC, Santa Catarina; SP, São Paulo.
Antimicrobial resistance
Table 2 shows that resistance to nalidixic acid (NAL), ampicillin (AMP), cefotaxime (CTX), ceftazidime (CAZ), ciprofloxacin (CIP) and tetracycline (TET) was the most prevalent in both sampling years and there was a significant increase in resistance to these antibiotics in the most recent survey. Resistance of the two prevalent species (Salmonella ser. Heidelberg and Salmonella ser. Minnesota) increased markedly from approximately 60% of isolates in 2014 to >85% in 2017 (Fig. 3). Conversely, there was a notable decrease in the rate of resistance to chloramphenicol in the second sampling period. None of the isolates was resistant to azithromycin or meropenem. Overall, in 2014, 17.8% (26/146) were susceptible to all antimicrobials tested compared with only 6.1% (10/163) in 2017. Multidrug resistance (MDR) was found in 50.7% (74/146) of the isolates from 2014, and this increased to 77.3% (126/163) in 2017 (P < 0.05). The highest MDR rates (3–6 antimicrobial classes) were evident for Salmonella ser. Heidelberg and Salmonella ser. Minnesota. Among the former serotype, MDR rates increased from 78.2% (43/55) in 2014 to 93.3% (83/89) in 2017, and for the latter from 64.7% (11/17) in 2014 and 86.8% (33/38) in 2017.

Fig. 3. Main resistance rates of the serotypes Heidelberg and Minnesota from poultry meat in Brazil in each year analysed.
Table 2. Prevalence of antimicrobial-resistant among Salmonella enterica from poultry meat in Brazil

CI, confidence interval; AMP, ampicillin; AZM, azithromycin; CAZ, ceftazidime; CHL, chloramphenicol; CIP, ciprofloxacin; CST, colistin; CTX, cefotaxime; GEN, gentamicin; MEM, meropenem; NAL, nalidixic acid; SXT, sulfamethoxazole/trimethoprim; TET, tetracycline; TGC, tigecycline.
Of note, the resistance profile NAL-AMP-CTX-CAZ-CIP-TET was the most prevalent in both periods, and accounted for 26.0% (38/146) in 2014, and 63.2% (103/163) in 2017. In the earlier sampling period, the great majority 89.5% (34/38) of isolates with this resistance profile were of the serotype Heidelberg, while in 2017, there was a marginal decrease in the prevalence of the latter to 70.9% (73/103) while 25.2% (26/103) were Salmonella ser. Minnesota. Combined resistance to ciprofloxacin and cefotaxime was detected in 37.0% (54/146) of the isolates in 2014, and 72.4% (118/163) in 2017; this was also mainly associated with the Heidelberg and Minnesota serotypes.
Discussion
This survey evaluated non-typhoidal Salmonella isolates from the main states involved in Brazilian poultry production, which together account for more than 95% of chicken meat production in the country [8]. Several different S. enterica serotypes were identified by automated ribotyping, which has been reported to give data consistent with conventional serotyping [Reference Bailey12] and has been used routinely by MAPA laboratories to determine Salmonella serovars since 2007. The two most frequent serotypes, among 34 different types (Table S1), identified in both sampling periods (2014 and 2017), were Salmonella ser. Heidelberg and Salmonella ser. Minnesota. Earlier studies from Brazil also identified Salmonella ser. Heidelberg from poultry carcasses and products in rates varying from 0.8% to 5% in isolates from 2007 to 2011 [Reference Costa17]. More recently, this serovar was found to be the most frequent (29.1%) by Fitch et al. [Reference Fitch18] in poultry meat, being found in three states in Brazil. This increasing trend is confirmed by the current study where it accounted for over one-third and one-half of all isolates, respectively, in the two sampling periods, and from an increased number of states. In the earlier period, isolates of this serovar were restricted to the Southern and Southeast regions of Brazil but by 2017 it had spread through the Southeast and to Midwest regions (Fig. 2).
Our results indicated that Salmonella ser. Minnesota is the second most common serotype in poultry carcasses in Brazil, in both 2014 and 2017, accounting for 11.6% (17/146) and 23.3% (38/163) of isolates, respectively. This serotype had already been reported as one of the five most prevalent in poultry carcasses from Brazil between 2007 and 2011, in rates varying from 9.1% to 40.24% [Reference Costa17–Reference Voss-Rech19]. Despite the similar prevalence to previous reports, this survey shows clear evidence of the spread of this serotype among the states producing broiler chickens in Brazil, as in 2014, it was identified in six states (SC, PR, MG, GO, MS, MT), while in 2017, it was also identified in ES, PA and SP, encompassing all regions related to chicken meat production in Brazil (Fig. 2).
Salmonella ser. Heidelberg is infrequently reported in human and animal sources from European countries [Reference Campos20]. However, it is the second most prevalent serotype in retail poultry meat in Colombia [Reference Donado-Godoy21]. In the USA and Canada, it is among the top five serotypes from poultry and is frequently associated with invasive human infections [Reference Foley22, 23]. Conversely, Salmonella ser. Minnesota has not been significantly associated with human infections and is rarely identified in animals in countries other than Brazil [Reference Silveira24]. The shift in the predominant S. enterica serotypes that occurred in recent years in Brazil is probably associated with the implementation of the control of Salmonella ser. Enteritidis and Salmonella ser. Typhimurium in poultry farms by the Brazilian Ministry of Agriculture in 2003 [25]. The decline in Salmonella ser. Enteritidis, which was the most prevalent serovar in poultry isolates from Brazil until then, would have allowed the occupation of this ecological niche by other serovars, such as Salmonella ser. Heidelberg and Salmonella ser. Minnesota. A similar phenomenon has occurred in the USA, where Salmonella ser. Heidelberg and Salmonella ser. Kentucky supplanted Salmonella ser. Enteritidis as the predominant serotypes in poultry [Reference Costa17, Reference Foley22].
Salmonella enterica isolates in this study displayed high and increasing rates of resistance, as over 80% were resistant to at least one antibiotic in 2014, and this rose to 94% in 2017. This change was likely due to the increase of MDR strains of Salmonella ser. Heidelberg and Salmonella ser. Minnesota (Fig. 3).
In 2014, the highest rate of resistance was to tetracycline (60.3%) which increased to 82.8% in 2017 (Table 2). Although tetracyclines have been prohibited for use as zootechnical additives since 1998 in Brazil [26], this class of antimicrobial was one of the first used in animal production and remains approved for therapeutic purposes. Voss-Rech et al. [Reference Voss-Rech19] also found high levels of resistance to tetracycline (52.4%) in broiler farms in Brazil. Similar results were reported in the USA (53.2% in poultry meat between 2008 and 2017) [Reference Yin27], Canada (44% in broiler chicken between 2013 and 2018) [Reference Caffrey28] and in countries of the European Union (46.1% in meat from broiler in 2016) [29].
Fluoroquinolones are critically important antimicrobials for human medicine [30] and are the drug of choice to treat invasive salmonellosis in adults [Reference Nair, Venkitanarayanan and Johny31]. Our results indicated high rates of resistance to nalidixic acid and ciprofloxacin among Salmonella isolates from poultry meat. Similar results were also recorded for Salmonella in broiler meat from the European Union, with rates in 2016 of 61.5% of resistance for nalidixic acid and 64.7% for ciprofloxacin [29]. Similarly, Colombia reported resistance rates among Salmonella of 66.0% for nalidixic acid and 41.2% for ciprofloxacin [Reference Donado-Godoy21], while in China 99.5% of isolates from broiler chickens were resistant to nalidixic acid, and 48.7% to ciprofloxacin [Reference Zhu32]. Voss-Rech et al. [Reference Voss-Rech33], in a meta-analysis study of the temporal evolution of AMR of non-typhoidal Salmonella from humans and poultry in Brazil, had already reported significantly increased levels of resistance to nalidixic acid in humans and poultry isolates, which may be an indicator of emerging resistance to fluoroquinolones in general [34]. On the other hand, fluoroquinolone resistance has been consistently low in Salmonella isolates from broiler chicken in Canada [Reference Caffrey28, Reference Romero-Barrios35] and in conventionally produced poultry meat in the USA [Reference Yin27], where these agents are not approved for use in poultry [Reference Mainali36, 37]. However, it is important to highlight that the European breakpoint for ciprofloxacin resistance (MIC >0.06 mg/l) is significantly more conservative than that in the USA (MIC >0.5 mg/l). Considering the MIC distribution (data not shown), 56.8% of our isolates from 2014 would be classified as having intermediate susceptibility to ciprofloxacin and none as resistant, according to Clinical and Laboratory Standards Institute (CLSI) breakpoints. In 2017, only two isolates would be considered as resistant, but the great majority would be classed as the intermediate phenotype.
Resistance to extended spectrum cephalosporins is also a serious concern, since these are the antibiotics of choice for treating invasive salmonellosis in children [Reference Nair, Venkitanarayanan and Johny31]. Our results indicated high and increasing rates of resistance to the third-generation agents, cefotaxime and ceftazidime (Table 2). In Brazil, ceftiofur (a third-generation cephalosporin used exclusively for veterinary medicine) is used to combat early mortality due to infection in chicks, a practice which may promote the selection of resistant strains [Reference Costa17]. Rates of resistance to ceftiofur and ceftriaxone of Salmonella ser. Heidelberg obtained from retail chicken carcasses were reported as 43.8% and 75.0%, respectively [Reference Medeiros38]. In the USA, resistance to ceftriaxone was recently detected in Salmonella after the approval of ceftiofur for use in livestock and poultry. This led to the limitation of the use of cephalosporins in food production in the USA and in several other countries [Reference McDermott, Zhao and Tate39]. Notably, in Canada, resistance to third-generation cephalosporins, which was mainly associated with the serovar Heidelberg in retail chicken meat, decreased from 21.0% in 2014 to 6.6% in 2016, in Salmonella isolates from chicken meat, after the industry banned the use of ceftiofur in broiler chickens in mid-2014 [34].
Combined resistance to fluoroquinolones and third-generation cephalosporins also deserves special attention, owing to the restriction of treatment options for human salmonellosis with combined resistance. In our study, 37.0% of the isolates from samples in 2014 were resistant to both ciprofloxacin and cefotaxime rising to 72.4% in 2017. This resistance phenotype was mainly associated with Salmonella ser. Heidelberg, which accounted for 72.2% of the isolates in 2014, and 68.6% in 2017. However, 12 different serotypes also showed this combined resistance profile, including Salmonella ser. Minnesota and Salmonella ser. Typhimurium. It is noteworthy that among countries of the European Union, this pattern of resistance is very rare, and was previously found only in two Salmonella spp. isolates from broiler meat in Belgium, in 2016 [29].
Resistance to polymyxins has regained importance in recent years due to the increasing number of infections caused by carbapenemase-producing Enterobacteriaceae, thereby limiting treatment options, and the description of transmissible colistin resistance mediated by the mcr−1 gene [Reference Liu40]. Colistin has been used for decades in veterinary medicine and in agricultural production, including as a growth promoter [Reference Poirel, Jayol and Nordmann41]. In Brazil, colistin was prohibited as a zootechnical additive in November 2016 [42], and we found low rates of resistance to this agent in both test periods (Table 2). However, the mcr-1 gene has been documented in Salmonella isolates from pork [Reference Rau43] and poultry meat [Reference Moreno44, Reference Rau45] in Brazil, highlighting the importance of monitoring resistance to colistin in the food chain. In Europe, resistance to colistin was reported by five countries in Salmonella isolates from broiler meat in 2016, in rates varying from 1.2% to 16.7% [29]. In the USA, colistin is not marketed or available for use in food-producing animals, and the national surveillance programme (NARMS) do not routinely determine susceptibility to the agent [Reference McDermott, Zhao and Tate39].
Regarding other clinically important antibiotics, our study showed very high rates of resistance to ampicillin in both periods (Table 2), which is one of the oldest antibiotics used in veterinary medicine [Reference Voss-Rech33]. Similarly, high ampicillin-resistant rates were recorded in Salmonella isolates from chicken carcasses in Brazil (38.0%) [Reference Medeiros38], China (87.8%) [Reference Zhu32], Turkey (85.2%) [Reference Yildirim46] and Mexico (82.9%) [Reference Miranda47]. On the other hand, Canada reported declining trends in resistance to ampicillin from 2011 (31.6%) to 2016 (7.1%) [34].
We found no resistance to azithromycin or meropenem among the study isolates. Similar findings for meropenem were reported by countries of the European Union, and azithromycin resistance was minimal [29]. Likewise, our survey identified only low levels of resistance to gentamicin, trimethoprim/sulfamethoxazole and tigecycline, with no trends of increase, while resistance to chloramphenicol decreased significantly over the assessed periods (Table 2). Data from Canada and the USA on Salmonella isolates from poultry showed similarly low rates of resistance for these antibiotics [Reference Romero-Barrios35, 48], except for tigecycline which is not tested by the US authorities for Salmonella isolates. In Europe, reported tigecycline resistance rates in 2016 varied from 1.2% to 16.7% from broiler meat [29].
In conclusion, our data provide important baseline information on the serotype distribution and AMR of non-typhoidal Salmonella isolates from poultry meat in Brazil. Such data should prove of value for the development and implementation of an Integrated Surveillance Program on Antimicrobial Resistance in Foodborne Pathogens in Brazil. Salmonella ser. Heidelberg and Salmonella ser. Minnesota were clearly the most frequent serotypes in the two survey periods. High and increasing rates of resistance were recorded for nalidixic acid, ampicillin, cefotaxime, ceftazidime, ciprofloxacin and tetracycline. These results stress the importance of continuous monitoring of AMR in the poultry food chain and the need to expand this surveillance to other food production animals.
Supplementary material
The supplementary material for this article can be found at https://doi.org/10.1017/S0950268821002156.
Financial support
This work was supported by the Brazilian Ministry of Agriculture, Livestock and Food Supply (MAPA), by ‘Instituto Nacional de Pesquisa em Resistência a Antimicrobianos’ (INPRA)and by “Fundo de Incentivo à Pesquisa e Eventos do Hospital de Clínicas de Porto Alegre” (FIPE/HCPA) (Project n° 2017-0541).
Conflict of interest
None.
Data availability statement
The authors confirm that the data supporting the findings of this study are available within the article and its Supplementary materials.