Introduction
Agroforestry systems are defined as land-use systems where woody perennials are managed together with crops and/or animals, and where ecological and economic interactions exist among the components as a result of spatial and temporal arrangements (Solis et al., Reference Solis, Vallejos-Torres, Arévalo, Marín-Díaz, Ñique-Alvarez, Engedal and Bruun2020). Coffee agroforestry technology is a traditional and complex agroforestry system in which coffees are cultivated under the shade of tall-growing plants (Abebe, Reference Abebe2005; Ehrenbergerová et al., Reference Ehrenbergerová, Šenfeldr and Habrová2017; Waktola and Fekadu, Reference Waktola and Fekadu2021). Coffee agroforestry systems provide a variety of ecosystem services, including biodiversity conservation (Mbow et al., Reference Mbow, Van Noordwijk, Luedeling, Neufeldt, Minang and Kowero2014a, Reference Mbow, Smith and Skole2014b; Venter, Reference Venter2014), an alternative source of income to address food security (Häger, Reference Häger2012; FAO, 2013), and mechanisms of carbon storage in both living biomass and soil (Bonan, Reference Bonan2008; Powlson et al., Reference Powlson, Whitmore and Goulding2011b). Furthermore, coffee agroforestry has emerged as a promising land-use system for reducing or offsetting deforestation (Soto-Pinto et al., Reference Soto-Pinto, Anzueto, Mendoza, Ferrer and de Jong2010). This is because coffee provides an alternative source of income that would otherwise be derived from forest cutting (Zomer et al., Reference Zomer, Neufeldt and Xu2016). The inclusion of trees within coffee farming systems has been shown to regulate the microclimate for coffee plants, add nutrients to the soil, improve soil properties and increase carbon storage (Alegre et al., Reference Alegre, Lao, Silva and Schrevens2017; Dollinger and Jose, Reference Dollinger and Jose2018).
Several studies have found that coffee agroforestry systems store substantially more carbon than sun-grown coffee, maize and other traditional mono-cropping systems (Dossa et al., Reference Dossa, Fernandes, Reid and Ezui2008; Tadesse et al., Reference Tadesse, Zavaleta and Shennan2014; Vanderhaegen et al., Reference Vanderhaegen, Verbist, Hundera and Muys2015; Betemariyam et al., Reference Betemariyam, Negash and Worku2020). Globally, coffee agroforestry systems have an estimated carbon sequestration potential of 44.8–466.5 Pg C/yr (in biomass and soil) (Nair et al., Reference Nair, Nair, Kumar and Showalter2010). In tropical agroforestry systems, carbon sequestration potential is estimated to be 95 t C/ha; varying widely between 12 and 228 t C/ha (Albrecht and Kandji, Reference Albrecht and Kandji2003). A global meta-analysis report showed that shaded perennial agroforests had 48% higher soil organic carbon (SOC) stocks than monoculture systems (Chatterjee et al., Reference Chatterjee, Nair, Chakraborty and Nair2018). The high carbon sequestration potential of the coffee agroforestry system is attributed to the availability of a diverse range of plant species (Mbow et al., Reference Mbow, Van Noordwijk, Luedeling, Neufeldt, Minang and Kowero2014a, Reference Mbow, Smith and Skole2014b). Since the adoption of the Kyoto Protocol in 1997, agroforestry has gained attention as a strategy to store carbon in agricultural landscapes and mitigate the effects of climate change (Jose and Bardhan, Reference Jose and Bardhan2012). As a result, shade-grown coffee systems have been recognized as viable afforestation and reforestation strategies for enhanced carbon sequestration and climate change mitigation.
Shade trees are commonly used in coffee plantations by farmers in the tropics (Dossa et al., Reference Dossa, Fernandes, Reid and Ezui2008; Solis et al., Reference Solis, Vallejos-Torres, Arévalo, Marín-Díaz, Ñique-Alvarez, Engedal and Bruun2020). In Ethiopia, coffee is grown in a traditional way following the principles that Lammerts van Bueren and Struik (Reference Lammerts van Bueren and Struik2004) referred to as ‘the concept of naturalness’, enhancing organic coffee production systems. This indicates that coffee plants are mainly grown in the forest or in farming systems that incorporate specific shade plants – usually indigenous trees, or sometimes fruit trees with high biomass components in the system (Valencia et al., Reference Valencia, Garcia-Barrios, West, Sterling and Naeem2014). Coffea arabica L. – a species native to Ethiopia, originating in the south-western part of the country is widely grown under different shade trees following different management practices (Bertrand et al., Reference Bertrand, Alpizar, Lara, SantaCreo, Hidalgo, Quijano, Montagnon, Georget and Etienne2011). The compatibility of shade tree species with coffee plants influences the potential carbon sequestration and yield of the coffee plants.
The existing attitude of coffee growers and land managers towards coffee plants diverges more towards its economic benefit. However, the vast area of coffee forests around the world plays a novel role in carbon sequestration and climate change mitigation. Many studies conducted to estimate the carbon stocks of the coffee agroforestry systems did not show the contribution of coffee plants to the total carbon stocks in coffee forests. This means that the percent share of coffee plants in storing carbon within coffee agroforestry systems was less empathized. Therefore, the aim of the study was to quantify the carbon sequestration potential of coffee agroforestry systems and estimate the contribution of coffee plants to the overall carbon stock of coffee agroforestry systems.
Materials and methods
Study area
The study was conducted in the Nono Sale district, which is located in Ilubabor zone, southwestern Oromia, Ethiopia. Geographically, it is situated between 7° 27′ and 8° 18′ N latitudes and 34° 52′ and 35° 26′ E longitudes, with an elevation range of 1300–2552 m above sea level. The mean annual rainfall ranges from 1700 to 2200 mm. The mean annual minimum and maximum temperature are 10 and 27°C, respectively (NMA, 2020). The total area of the district is 215 550 ha, out of which 62 684 ha (29% of the district) are occupied by the coffee agroforestry systems. The present study covered 105 ha of coffee agroforestry systems where coffee plants are commonly grown under shade trees. The most common shade tree species for C. arabica L. in the study area include peacock flower (Albizia gummifera), large-podded albizia (Albizia schimperiana), waterberry (Syzygium guineense), croton (Croton macrostachyus), ‘Birbira’ (Millettia aferruginea), Sudan teak (Cordia Africana), ‘Kosso’ (Schefflera abyssinica) and many other indigenous tree species. Some sections of the coffee agroforestry systems had naturally mixed tree species with no species dominance, whereas others were dominated by A. gummifera or S. guineense.
Stratification and sampling techniques
The coffee agroforestry systems in the study area were classified into three strata based on shade tree species dominance and the coffee management system. Accordingly, the first stratum covers 51 ha of mixed trees-shaded coffee forest with a semi-natural coffee management system; the second stratum covers 34 ha of A. gummifera-dominated coffee forest with the semi-natural coffee management system, and the last stratum consists of 20 ha of S. guineense-dominated coffee forest with a natural coffee management system. A natural coffee management system is a management system in which coffee grows naturally in primary forests without the interventions of humans. This system is referred to as wild coffee farming. A semi-natural coffee management system is a system whereby coffee is partly managed by humans. However, the semi-natural coffee management system is not as intensively managed as plantation coffee.
Sample plots were laid in the coffee agroforestry strata using a purposive sampling technique. In each stratum, nested sample plots of 20 × 20 m, 2 × 2 m and 1 × 1 m were laid to measure the biomass of woody plants, herbaceous plants and litter fall, respectively. Based on UNFCCC (2010) sample estimation guideline, a total of 34 sample plots were surveyed in the study area (17 sample plots for mixed trees-shaded coffee forest stratum, 11 for Albizia-dominated coffee stratum and 6 for Syzygium-dominated coffee stratum). The biomass of coffee as well as all other trees and shrubs in the sample plots were measured following standard techniques. Soil samples were collected from 0–30 to 30–60 cm depths at four corners and centre of the main plot in each stratum and mixed to make a composite sample for each layer in the plot.
Estimation of coffee forest biomass
Aboveground biomass (AGB)
In the larger plot, diameter at breast height (DBH) (i.e. at 1.3 m) and tree height (H) of each shade tree were measured with a caliper and hypsometer, respectively. The wood-specific gravity (density) was obtained at the species level from the global wood density database (Chave et al., Reference Chave, Coomes, Jansen, Lewis, Swenson and Zanne2009). The aboveground biomass of living shade trees with DBH ≥ 5 cm was then estimated using the revised allometric equation developed by Chave et al. (Reference Chave, Rejou-Mechain, Burquez, Chidumayo, Colgan, Delitti, Duque, Eid, Fearnside and Goodman2014) for tropical forest stands (Eqn (1)):

where AGBST is the aboveground biomass of shade trees (kg), ρ is the wood specific gravity (g/cm3), DBH is the diameter at breast height (cm) and H is the tree height (m). After measuring all coffee stamps with a diameter ≥3.8 cm at 40 cm height in the main plots, the AGB of coffee plants was estimated using the allometric equations developed by Negash et al. (Reference Negash, Starr, Kanninen and Berhe2013) (Eqn (2)):

where AGBCP is the aboveground biomass of coffee plants (kg/plant), d 40 is the diameter of coffee plants at 40 cm. Then, the total AGB was derived by adding the AGBST and AGBCP.
Belowground biomass (BGB)
Based on the root-to-shoot ratio of 1:5 for trees developed by MacDicken (Reference MacDicken1997), BGB is 20% of the aboveground tree biomass. Thus, the BGB of shade trees was estimated using Eqn (3):

where BGBST is the belowground biomass of shade trees (kg/plant), AGBST is expressed in kg/plant and 0.2 is the conversion factor. On the other hand, the BGB of coffee plants was estimated based on the root-to-shoot ratios developed by Kuyah et al. (Reference Kuyah, Dietz and Muthuri2012) and Negash et al. (Reference Negash, Starr, Kanninen and Berhe2013) (Eqn (4)):

where BGBCP is the belowground biomass of coffee plants (kg/plant), AGBCP is expressed in kg/plant. Hereafter, the total BGB was estimated as a summation of the BGBST and BGBCP.
Herbaceous/saplings biomass
Non-woody aboveground live vegetation that has less than 5 cm DBH was considered as grasses and herbaceous biomass. The frame sampling method (i.e. 2 × 2 m frame) was used to measure the biomass of herbaceous and grasses. The frames were laid at the four corners and centre of the main sample plot, and all living herbaceous inside the frame was cut at base to record the fresh weight, from which a 500 g sample size was taken to the laboratory and oven-dried at 85°C to measure the dry weight.
Litter biomass
The dry matter of litter and finer plant debris was collected from 1 × 1 m plot in every four corners and centre of the main plot in the nest. Litter was collected in 1 m2 plots and the total fresh weight was recorded, after which a 250 g sample size was taken to the laboratory, oven-dried at 85°C, and reweighed to estimate the dry matter.
Estimation of carbon from biomass
The amount of carbon sequestered (Cx in kg) in the shade trees, herbaceous and litter fall was determined by multiplying the biomass of each pool by 0.50 (Chave et al., Reference Chave, Rejou-Mechain, Burquez, Chidumayo, Colgan, Delitti, Duque, Eid, Fearnside and Goodman2014) using Eqn (5):

According to Kuyah et al. (Reference Kuyah, Dietz and Muthuri2012) and Negash et al. (Reference Negash, Starr, Kanninen and Berhe2013), the amount of carbon stored in the coffee plants was obtained by multiplying 0.49 to the biomass of coffee plants using Eqn (6):

The total amount of carbon stored in biomasses per plot was then calculated by adding the carbon stored in each biomass pool for all plant species and litter fall in a plot.
Soil analysis and estimation of SOC stocks
Soil samples collected from 0‒30 to 30‒60 cm layers in the 34 sample plots were air dried and crushed to pass through 2 mm sieve size. Bulk density was determined using core method (Blake and Hartge, Reference Blake, Hartge and Klute1986), whereas SOC content was determined using Walkley–Black oxidation method (Walkley and Black, Reference Walkley and Black1934). Consequently, SOC stock (t C/ha) in a specific soil depth was estimated using Eqn (7) as:

where OC is the organic carbon (mg C/g soil), d is the soil thickness or depth (i.e. 0–30 cm and 30–60 cm), ρb is the bulk density of the soil (g/cm3).
In general, the overall total carbon sequestered per plot was estimated by adding the total carbon sequestered in plants biomass and in soil.
Estimation of equivalent CO2 sink
As 1 t of carbon is equal to 3.67 t of CO2, the total equivalent CO2 sink (t) in the coffee agroforestry systems was estimated using Eqn (8):

where CO2e is the carbon-dioxide equivalent, TC is the total carbon and 3.67 is the conversion factor.
Statistical data analysis
The results were subjected to analysis of variance using R version 4.0.3 software as well as XLSTAT Version 2017 software. Principal component analysis was used to see the relationship between components of carbon. The least significant difference of means was computed at P < 0.05.
Results
Carbon storage capacity of coffee agroforestry systems
The estimates of carbon stocks of Albizia-shaded coffee stratum, Syzygium-shaded coffee stratum, and mixed trees-shaded coffee stratum indicate that carbon sequestration in the coffee agroforestry systems varied with coffee management system and the type of shade tree (Table 1). The total carbon stock of coffee agroforestry systems ranged from 254.9 t C/ha in the Syzygium-shaded stratum to 321.8 t C/ha in the Albizia-shaded stratum (Table 1). Mixed trees and Albizia-shaded strata received a semi-natural coffee management system (i.e. a better management practice) and thus stored more carbon than Syzygium-shaded stratum that received a natural coffee management system. The overall mean carbon sequestered in the coffee agroforestry systems was 287.1 t C/ha.
Table 1. The carbon storage potential of coffee agroforestry systems by carbon components
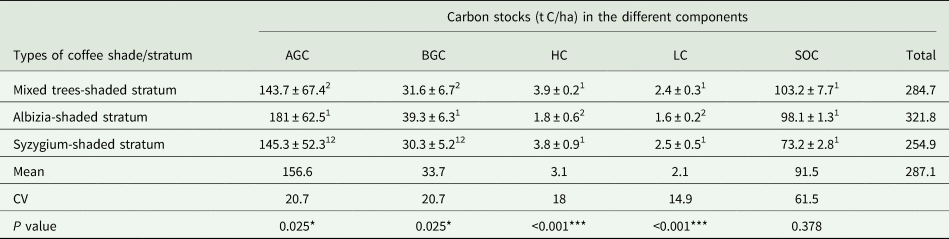
AGC, aboveground carbon; BGC, belowground carbon; HC, herbaceous carbon; LC, litter carbon; SOC, soil organic carbon.
SOC was measured to a depth of 60 cm. Means followed by different superscript numbers in the same column are significantly different (*** at P < 0.001, ** at P < 0.01 and * at P < 0.05).
The mean carbon storage capacity of different carbon pools ranged from 2.1 t C/ha in the LC to 156.6 t C/ha in the AGC (Table 1). The total carbon storage capacity (t C/ha) of different carbon components decreased in the following order: AGC > SOC > BGC > HC > LC. The AGC component had the highest C stock in the coffee agroforestry systems. We found SOC stocks ranging from 73.2 t C/ha for the Syzygium-shaded coffee stratum to 103.2 t C/ha for the mixed trees-shaded coffee stratum to a depth of 60 cm (Table 1). The relatively high SOC stock in the mixed trees-shaded stratum could be due to the presence of various plant species that have different degrees of decomposition. The SOC showed a higher coefficient of variation (CV = 61.5%) followed by AGC (CV = 20.7%) and BGC (20.7%) (Table 1), indicating greater spatial variability of carbon stock in soils than vegetation. The mean estimates of AGC and BGC in the mixed trees-shaded coffee stratum were significantly different from that of the Albizia-shaded coffee stratum at P < 0.05 (Table 1). They also significantly differ in their HC and LC contents at P < 0.001. However, there was no significant difference between carbon sored in the respective carbon components of mixed trees-shaded stratum and Syzygium-shaded stratum at P < 0.05. Similarly, SOC estimates did not differ significantly among the three strata at P < 0.05.
The proportions of carbon stored in the stratum and the entire coffee agroforestry system were affected by the interaction effect of the different types of coffee shade and carbon components. The coffee stratum shaded by Albizia had sequestered 56.3% of carbon in the AGC, 30.5% in the SOC, 12.2% in the BGC and the rest in the HC and LC (Fig. 1). Similarly, the coffee stratum shaded by mixed trees species had sequestered 50.5, 36.2, 11.1, 1.4 and 0.8% in the AGC, SOC, BGC, HB and LB, respectively (Fig. 1). The results indicate that the highest proportion of carbon was stored in the AGC pool followed by SOC pool, whereas the lowest proportion of carbon was stored in the litter pool in all strata. The mean percentage of carbon stored in the different carbon components ranged from 0.8% in the LC to 54.6% in the AGC.

Fig. 1. Colour online. The percentage proportion of carbon stored in the different stratum and carbon components. The carbon components include AGC, aboveground carbon; BGC, belowground carbon; HC, herbaceous carbon; LC, litter carbon; SOC, soil organic carbon.
Net carbon sequestration potential of coffee plants
Coffee plants contributed significantly to the total carbon stock of coffee agroforestry systems. The net carbon sequestered by coffee plants in the coffee agroforestry systems was 48.5, 45 and 18.8 t C/ha in the Albizia-shaded coffee stratum, mixed trees-shaded coffee stratum and Syzygium-shaded coffee stratum, respectively (Table 2). Coffee plants contributed nearly 15.8, 15.1 and 7.4% of the carbon sequestered in the mixed trees-shaded coffee stratum, Albizia-shaded coffee stratum and Syzygium-shaded coffee stratum, respectively (Table 2). The overall mean carbon sequestered by coffee plants in the coffee agroforestry systems of the present study area was 37.5 t C/ha. On average, 12.8% of the carbon sequestered in the coffee agroforestry systems was augmented by coffee plants.
Table 2. The amount and percentage proportion of carbon sequestered by coffee plants in the coffee agroforestry systems
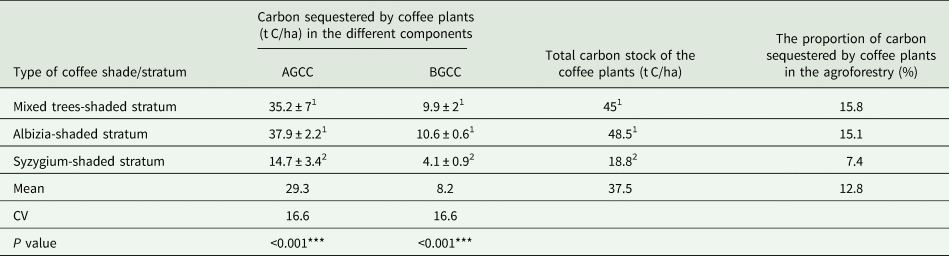
AGCC, aboveground coffee carbon; BGCC, belowground coffee carbon.
Means followed by different superscript numbers in the same column are significantly different (*** at P < 0.001).
The results show that the biomass of coffee plants was more vigorous in the Albizia-shaded stratum followed by the mixed trees-shaded stratum and the Syzygium-shaded stratum. A single Albizia tree has a large canopy that can shade up to 30 coffee plants, whereas a single Syzygium tree can only shade up to six coffee plants. The diversity of tree species in the mixed trees stratum, on the other hand, may have created suitable soils and environments for the growth of coffee plants. Furthermore, the coffee plants grown in the Albizia and mixed-tree-shaded coffee strata are managed using a semi-natural coffee management system, implying that the semi-natural coffee management system produces more biomass than the natural coffee management system. The mean values of carbon sequestered by coffee plants in the Albizia-shaded and mixed trees-shaded strata were significantly different from that of the Syzygium-shaded stratum at P < 0.001, but they did not significantly differ from each other (Table 2).
The implication of coffee agroforestry to climate change mitigation
In the study area, coffee agroforestry systems sequestered a large amount of carbon in the vegetation including coffee plants and soils. Proportional to the area of each stratum, approximately 14.5, 10.9 and 5.1 Gg C were stored in the mixed trees-shaded stratum, Albizia-shaded stratum and Syzygium-shaded coffee stratum, respectively (Table 3). This shows that a total of 30.5 Gg C was stored in the entire coffee agroforestry systems of the study area. Particularly, coffee plants added a total of 4.4 Gg C into the coffee agroforestry systems (Table 3).
Table 3. Total carbon stock and equivalent carbon-dioxide (CO2) sink across different coffee agroforestry systems and coffee plants

*1 Gg = 1000 tons.
The entire coffee agroforestry systems of the present study area trapped 111.9 Gg CO2 from the atmosphere, whereas coffee plants trapped 16.1 Gg CO2 from the atmosphere and stored it as carbon in the agroforestry systems. Apart from its economic potential, coffee plants play a great role in storing carbon and regulating climate change. Therefore, the economic returns from coffee plants should not be expressed only in terms of their yield but also in the credit that could be obtained by carbon sequestration.
Relationship among carbon components
The relationship between the carbon components of coffee agroforestry systems was described using a factor plot (Fig. 2). Here, the components of carbon were displayed on the two-dimensional space using the first two components. There was a strong correlation between HC and LC as observed from the magnitude and direction of correlation lines (Fig. 2). This implies that the amount of LC increases as herbaceous biomass increases. There was also a positive correlation between SOC and coffee carbon (CC) (Fig. 2). When the amount of SOC increases, the fertility of soils improves. As a result, coffee plants grown on fertile soils produce high biomass and sequester a considerable amount of carbon. The amount of BGC depends on the AGC. They have direct relationships as presented in Fig. 2. The mixed trees-shaded stratum showed relatively better HC and LC whereas the Albizia-shaded stratum showed better AGC and BGC than other strata (Fig. 2).

Fig. 2. Colour online. Factor plot of the first two principal components. The factor loading shows the relationship between the components of carbon in the coffee agroforestry systems. The components of carbon include AGC, aboveground carbon; BGC, belowground carbon; HC, herbaceous carbon; LC, litter carbon; SOC, soil organic carbon.
Discussion
The carbon storage capacity of coffee agroforestry systems in the study area varied with the type of shade tree. Coffee carbon sequestration was highest in the Albizia-shaded coffee stratum, followed by the mixed trees-shaded coffee stratum and Syzygium-shaded coffee agroforestry systems. Albizia trees have a large canopy that can provide shade for many coffee plants. This demonstrates that A. gummifera was a suitable shade tree for coffee plants. On the other hand, the diversity of tree species in the mixed trees stratum could have also created suitable soils and environments for the growth of coffee plants. The tree species richness influenced carbon sequestration in both aboveground and belowground carbon pools. As a result, the carbon stock of coffee plants grown under various trees was used to judge and rank the suitability of native trees for coffee shade.
The mean carbon stock of coffee agroforestry systems varies across the globe. The carbon stocks (biomass plus soils) of coffee agroforestry systems in our study site (254.9–321.8 t C/ha, with an average of 287.1 t C/ha) were higher than those reported for low latitude (0–25°) forest ecosystems (mean 244 t C/ha; Dixon et al., Reference Dixon, Brown, Houghton, Solomon, Trexler and Wisniewski1994) and above the range reported for global agroforestry systems (12–228 t C/ha; Albrecht and Kandji, Reference Albrecht and Kandji2003). It was also higher than the mean carbon stocks reported for coffee agroforestry systems in different countries around the world, including 194.96 t C/ha in the Mana district of southwestern Ethiopia (Betemariyam et al., Reference Betemariyam, Negash and Worku2020), 259 t C/ha in Guatemala (Schmitt-Harsh et al., Reference Schmitt-Harsh, Evans, Castellanos and Randolph2020), 213.80 t C/ha in Mexico (Soto-Pinto et al., Reference Soto-Pinto, Anzueto, Mendoza, Ferrer and de Jong2010) and 82 t C/ha in Indonesia (van Noordwijk et al., Reference van Noordwijk, Rahayu, Hairiah, Wulan, Farida and Verbist2002). However, the upper limit of the carbon stock range in this study (321.8 t C/ha) was lower than the upper limit of the carbon stock range (377 t C/ha) reported by Negash and Starr (Reference Negash and Starr2015) for the south-eastern Rift Valley escarpment of Ethiopia.
The carbon sequestration potential of coffee agroforestry systems varied with the type of carbon pool. In this study, the aboveground pool had a higher carbon stock and the litter fall had a lower carbon stock than the other pools. The size of trees rather than the number of trees contributed most to carbon stocks in the aboveground biomass, showing that the incorporation of long-lived trees in agroforestry systems provides opportunities to increase carbon storage (Albrecht and Kandji, Reference Albrecht and Kandji2003). The mean SOC of coffee agroforestry systems in the present study (91.5 t C/ha) was lower than the mean SOC stock of 123.5 t C/ha to 60 cm depth reported by Solis et al. (Reference Solis, Vallejos-Torres, Arévalo, Marín-Díaz, Ñique-Alvarez, Engedal and Bruun2020) for shaded coffee in the Peruvian Amazon. The high SOC stocks in shaded systems are due to the high organic matter input from trees, which also maintain adequate litter layers to protect the soil surface (Bruun et al., Reference Bruun, De Neergaard, Lawrence and Ziegler2009; Hairiah et al., Reference Hairiah, van Noordwijk, Sari, Saputra, Widianto, Suprayogo, Kurniawan, Prayogo and Gusli2020). It has been widely recognized that practices that improve SOC stocks are important to ensuring the sustainability of soil functions (Lal, Reference Lal2004; Powlson et al., Reference Powlson, Gregory, Whalley, Quinton, Hopkins, Whitmore, Hirsch and Goulding2011a). High SOC stocks not only maintain soil quality and site productivity but also constitute a more permanent store of carbon than biomass (Negash and Starr, Reference Negash and Starr2015). This could be because biomass is easily converted to the labile pools of soil carbon within seasons or years. However, soil stores carbon in a passive or recalcitrant form that turns over only in hundreds to thousands of years.
The differences in carbon stocks observed in different parts of the world could be attributed to a variety of factors, including coffee species, differences in the allometric equations used, management practices and site factors such as climate and soil conditions. For instance, in the coffee agroforestry systems studied by Mohammed and Bekele (Reference Mohammed and Bekele2014), the diameter of coffee shrubs was measured at 15 cm above ground, whereas in our study, the diameter was measured at 40 cm height above ground. Furthermore, Mohammed and Bekele (Reference Mohammed and Bekele2014) estimated the aboveground biomass of trees and coffee plants in coffee agroforestry systems using allometric equations developed by Brown et al. (Reference Brown, Gillespie and Lugo1989) and Segura et al. (Reference Segura, Kanninen and Suárez2006), whereas we estimated the aboveground using the generic equation developed by Negash et al. (Reference Negash, Starr, Kanninen and Berhe2013) for coffee plants and Chave et al. (Reference Chave, Rejou-Mechain, Burquez, Chidumayo, Colgan, Delitti, Duque, Eid, Fearnside and Goodman2014) for other trees. Similar allometric equations could also provide different biomass estimates for the same coffee species and coffee agroforestry systems in different agroclimates. This is because the climatic condition of a given area governs the rate of photosynthesis and biomass formation in plants.
We investigated that farmers conserve many native tree species in the study area for coffee shade. As a result, coffee-based forests receive more attention compared to forests without coffee plants. Trees, shrubs and coffee plants in the coffee agroforestry systems get more protection from livestock and human pressure. The native trees were conserved to develop a high canopy and biomass for protecting the coffee plants. Studies show that in humid tropical landscapes, the coffee agroforestry system has a comparable conservation value to natural forests (Donald, Reference Donald2004; Bhagwat et al., Reference Bhagwat, Willis, Birks and Whittaker2008). This means that coffee agroforestry systems provide multiple functions, including the conservation of native tree species, the provision of habitat for other species, the creation of a biological corridor between protected areas, and the alleviation of resource-use pressure on conservation areas (McNeely and Schroth, Reference McNeely and Schroth2006; Bhagwat et al., Reference Bhagwat, Willis, Birks and Whittaker2008). As a result, the species richness and abundance, as well as the potential for carbon sequestration, were higher in coffee agroforestry than in natural forests without coffee.
Coffee agroforestry systems can provide a wide range of ecosystem services such as carbon sequestration and climate change regulation, soil erosion and fertility improvement and biodiversity conservation in fragmented landscapes (Vaast et al., Reference Vaast, Beer, Harvey and Harmand2005). Carbon sequestration is greatly enhanced by the existence of shade trees when compared to full sun coffee monoculture. Higher carbon storage potential is assumed to co-occur with biodiversity conservation (Venter, Reference Venter2014). Shade trees also help to reduce runoff, resulting in less soil erosion and increased nutrient availability in the soil. Shade trees offer numerous advantages; there is an added value of wood production, which can be expressed in terms of woody biomass or carbon fixation (Häger, Reference Häger2012; Hergoulac'h et al., Reference Hergoulac'h, Blancart, Skiba, Hénault and Harmand2012), and they also act as a critical refuge for forest biota, including birds, insects, mammals and reptiles (Moguel and Toledo, Reference Moguel and Toledo1999). Coffee is the most widely commercialized tropical crop (Da Silva and Leite, Reference Da Silva and Leite2013), and it provides a significant source of income for millions of farmers (Donald, Reference Donald2004). As a result, coffee improves farmers' income and reduces their reliance on forests, thereby reducing deforestation. All agroforestry systems has three main functions, namely regulating rainwater (soil and water conservation), sequestering carbon (reducing the impact of global warming) and supporting the microclimate (crop production) (Budiastuti et al., Reference Budiastuti, Purnomo and Setyaningrum2022). Inclusion of coffee plants in the agroforestry system plays important roles in fostering these functions. Use of a carbon approach or function when comparing the contribution of coffee plants in coffee agroforestry systems is important for sustainable landscape management. In general, our findings show that coffee agroforestry systems can sequester substantial amount of carbon by trapping CO2 from the atmosphere, aiding in the mitigation of climate change.
Conclusions
Coffee plants sequester substantial amounts of carbon in the coffee agroforestry systems. The carbon sequestration potential of shade-grown coffee plants is influenced by the compatibility of the shade trees. Coffee forests are better protected than non-coffee forests, and thus coffee plants can be considered the keys to sustainable forest management. Hence, we suggest the expansion of coffee forest farming and the initiation of coffee carbon credits as strategies to encourage farmers to grow more organic coffee plants, conserve shade trees and ultimately contribute to climate change mitigation.
Acknowledgements
We thank Bedele Soil Research Center for supporting us during the laboratory analysis. We also thank the Environment, Forest, and Climate Change Authority for providing the necessary equipments used to measure tree metrics in the field.
Author's contributions
G. N. conceived and designed the study, conducted data gathering, performed statistical analyses and wrote the article, B. I. designed the study and revised the article, G. K. and A. C. edited the article.
Financial support
This work was supported by the Ethiopian Ministry of Education with the host of Wollega University.
Conflict of interest
The authors declare that there is no conflict of interest.
Ethical standards
Not applicable.