Introduction
Elephant grass (Cenchrus purpureus Schum. syn. Pennisetum purpureum Schum.) is an important forage crop, largely used in cut-and-carry systems, due to its great persistence and productivity (Silva et al., Reference Silva, Sales, Lemos, Silva, Ribeiro, Santos, Mello and Cunha2021a). In general, tall varieties of elephant grass are preferred to be used under cut-and-carry systems, due to their incredible herbage accumulation. However, the species has a vast genetic variability, with varieties varying substantially in their morphological, productive and nutritional characteristics (Cunha et al., Reference Cunha, Lira, Santos, Freitas, Dubeux Junior, Mello and Martins2011; Gomide et al., Reference Gomide, Chaves, Ribeiro, Sollenberger, Paciullo, Pereira and Morenz2015; Pereira et al., Reference Pereira, Lédo and Machado2017). The use of dwarf elephant grass varieties has increased in recent decades, which were initially selected for their use in grazing systems, but also have been adopted in cut-and-carry systems (Silva et al., Reference Silva, Santos, Lira, Mello, Melo, Dubeux Junior, Ferreira and Carvalho2016; Souza et al., Reference Souza, Santos, Cunha, Gonçalves, Silva, Mello and Dubeux2021). Dwarf elephant grass varieties in comparison with tall ones display a greater leaf–stem ratio and number of leaves per tiller, which provide better nutritive value (Souza et al., Reference Souza, Faturi, Rodrigues, Silva Filho, Rêgo and Rodrigues Filho2017). Conversely, tall varieties display greater internode length and stem elongation, which in turn, ensure greater herbage accumulation (Silva et al., Reference Silva, Sales, Lemos, Silva, Ribeiro, Santos, Mello and Cunha2021a).
Elephant grass varieties are very demanding in terms of nutrients and are known to have requirements for N from 200 to 400 kg/ha per year (Na et al., Reference Na, Sollenberger, Erickson, Woodard, Vendramini and Silveira2015; Alves et al., Reference Alves, Carneiro, Edvan, Cândido, Furtado, Pereira and Nascimento2018). Nevertheless, considering the elevated costs of N inorganic fertilizers, other options to provide inputs of N in the systems are required. The use of intercropping systems of forage grasses and legumes is an option (Seibt et al., Reference Seibt, Olivo, Alessio, Silva, Quatrin and Anjos2018) as the legume inclusion in the system can increase the nitrogen input via biological fixation. The N transfer from legumes to grasses occurs in diverse pathways, such as increments of N in the soil solution, litter and root decomposition (Schultze-Kraft et al., Reference Schultze-Kraft, Rao, Peters, Clements, Bai and Liu2018). These inputs eventually reduce the costs of N fertilizers and potential environmental impacts such as leaching and contamination of water bodies by the excessive use of inorganic fertilizers. It also improves forage nutritional value by increasing protein content in comparison with grasses grown alone in cut-and-carry systems (Boddey et al., Reference Boddey, Casagrande, Homem and Alves2020; Costa et al., Reference Costa, Mello, Guim, Costa, Abreu, Silva, Silva and Simões Neto2022).
However, tropical grasses often display greater use efficiency of water and CO2 than forage legumes, besides more significant yield responses to fertilization, leading to legume suppression in grass–legume intercrops (Seibt et al., Reference Seibt, Olivo, Alessio, Silva, Quatrin and Anjos2018). The success of this type of consortium depends on the compatibility between the species, considering the interspecific competition for resources (e.g. water, soil nutrients and photosynthetically active radiation (PAR)). In this scenario, intercropping of elephant grass and butterfly pea (Clitoria ternatea L.) might be compatible, because of the difference in their growth habits, in which erect and sturdy stems of elephant grass provide support to the climbing stems of butterfly pea, while the legume contributes to the nitrogen fixation and enhances the nutritive fodder value (Seibt et al., Reference Seibt, Olivo, Alessio, Silva, Quatrin and Anjos2018).
The butterfly pea is a perennial tropical legume with moderate requirements in soil fertility, and productivity that can vary from 12 to 25 t/ha per year of dry matter (DM) under favourable conditions (Gomez and Kalamani, Reference Gomez and Kalamani2003; Abreu et al., Reference Abreu, Vieira, Rocha, Araújo, Glória, Fernandes and Júnior2014). The agronomic responses of butterfly pea allow for growing the legume in cut-and-carry systems, including when it is intercropped with grasses (Mohammed, Reference Mohammed2013; Oguis et al., Reference Oguis, Gilding, Jackson and Craik2019).
Nevertheless, the intercropping dynamics can vary substantially depending on a series of factors, especially related to the species used (e.g. plant canopy structures, plant statures and their spatial arrangement above the ground). Examination of these traits helps to understand the plant responses to ecological factors, and how these factors can influence both herbage accumulation and forage quality (Da Silva et al., Reference Da Silva, Sbrissia and Pereira2015). Furthermore, the spatial arrangement modulates the attenuation of PAR along the vertical canopy gradient (Guenni et al., Reference Guenni, Romero, Guédez, Guenni and Pittermann2018). In horizontal canopies, the plant architecture and leaf angle lead to lower light penetration to the bases of the plant or soil level, while in vertical ones, there is a better light distribution throughout the canopy. Thus, legume canopies tend to be horizontals with greater light extinction than those composed of grasses (Faurie et al., Reference Faurie, Soussana and Sinoquet1996).
This study hypothesized that dwarf elephant grass varieties have lower radiation use efficiency (RUE) than tall ones, because of the morphophysiological traits of their canopies, such as great leaf area index (LAI) combined with a considerable light extinction. Additionally, we believed that the elephant grass–butterfly pea intercropping does not change significantly the canopy structure and RUE of the elephant grass harvested under cutting management. Based on these premises, the objective of this study was to evaluate the RUE and the structure of canopies formed by elephant grass varieties of contrasting plant stature in combination with butterfly pea as a legume intercrop under cut-and-carry systems.
Materials and methods
The experiment was carried out at the Sugarcane Experimental Station of Carpina – Universidade Federal Rural de Pernambuco (SESC/UFRPE) located in Carpina (07°51′03′′S, 35°15′17′′W, and 180-m altitude), Pernambuco state, Brazil. The climate at the location is classified as dry tropical (Alvares et al., Reference Alvares, Stape, Sentelhas, Gonçalves and Sparovek2013). The historical average annual rainfall for the site is 1100 mm per year, while the historical average temperature is 24.6°C, according to the SESC data (Santos et al., Reference Santos, Freire, Oliveira, Barbosa, Moura, Lopes and Santos2018a). Monthly rainfall and temperature averages during the experimental period are shown in Fig. 1. The soil was classified as distrocohesive yellow argisol (Santos et al., Reference Santos, Jacomine, Anjos, Oliveira, Lumbreras, Coelho and Cunha2018b) or acrisol, according to the World Reference Base for Soil Resources (IUSS Working Group WRB, 2015).

Fig. 1. Monthly rainfall (bars), maximum (), mean (
) and minimum temperature (
) over 2019 and 2020 at the Sugarcane Experimental Station of Carpina – Universidade Federal Rural de Pernambuco (SESC/UFRPE), located in Carpina, Pernambuco, Brazil. Source: SESC/UFRPE and Water and Climate Agency of Pernambuco State.
The experimental design was a randomized complete block, in factorial arrangement (4 × 2) with four replicates (n = 4). Four elephant grass varieties were assessed: elephant B and IRI-381 (tall-types), and the dwarf types Mott and Taiwan A-146 2.37. Moreover, two cropping systems were studied: elephant grass under monocropping and intercropped with butterfly pea.
The experimental plots of elephant grass varieties were established in 2014, totalling 32 plots with 16 m2 size (4 × 4 m2), 1-m space between plot rows, and 8-m2 net plot area for sampling, formed by excluding the first and last rows. All varieties were established at the same time, using stem seedlings planted into 15-cm deep furrows. The seedlings of the tall varieties averaged 80 cm in length while those of the dwarf varieties were shorter, measuring approximately 40 cm. At the time of planting, the plots were fertilized with 80 kg/ha of K2O and 70 kg/ha of P2O5, using triple superphosphate (46% P2O5) and potassium chloride (60% K2O) as sources. Before our study, these elephant grass varieties were kept under harvesting management in another trial, from 2014 to 2017. During this time, doses of 100 kg/ha of N and 80 kg/ha of K2O were applied in each harvest of the rainy seasons, using urea (45% N) and potassium chloride as sources.
The establishment of the intercrops occurred only in the second half of 2018. The legume was planted in 16 of the 32 experimental plots, through a randomized selection within the experimental blocks. Thirty-six butterfly pea seedlings aged 60 days were transplanted to each plot, between the elephant grass rows and spaced apart 0.5-m each other. The legume seedlings were transplanted right after an elephant grass cut at ground level to give them better conditions for development in the intercropping system. The butterfly seeding rate was 2.85 kg/ha, and the establishment phase of the intercrops lasted 6 months, from August 2018 to February 2019.
The experimental period and data collection occurred from March 2019 to December 2020. The harvests were made between May and December of 2019 and 2020 (years 1 and 2), under a frequency of 60-day regrowth, an intensity of 20-cm stubble height for the butterfly pea, besides cuts at the ground level for the elephant grass varieties. Harvests made at 60-day regrowth are commonly recommended to reach high herbage accumulation plus a suitable nutritive value of elephant grass varieties in cut-and-carry systems implanted in tropical regions (Valadares Filho et al., Reference Valadares Filho, Silva, Gionbelli, Rotta, Marcondes, Chizzotti and Prados2016).
Eight harvests were carried out during the experimental period. Four of them occurred in 2019 on 20 May 2019, 20 July 2019, 20 September 2019 and 20 November 2019. Four other harvests were performed in 2020 on 4 June 2020, 4 August 2020, 4 October 2020 and 4 December 2020. The harvest frequency of 60 days was maintained throughout the experimental period.
Fertilizer was applied during the rainy seasons of 2019 and 2020 (April–September), according to the recommendations of Cavalcanti et al. (Reference Cavalcanti, Lima Júnior and Lima2008) and the following soil chemical properties: 0.05, 0.06, 1.60, 0.80, 0.00 and 2.40 cmolc/dm3 for K, Na, Ca, Mg, Al3+ and H + Al, respectively, plus 5 mg/dm3 of P (Mehlich-1) and 5.5 pH (H2O). The fertilizer rates of N, K2O and P2O5 were applied by 100, 60 and 70 kg/ha thrice in the first three harvests in 2019 and twice in the first two harvests in 2020. Fertilizer was applied as urea, potassium chloride and triple superphosphate. Plots composed of intercrops were fertilized only with K2O and P2O5 and did not receive any supplemental N.
All the fresh herbage accumulated every 60-day regrowth was harvested and weighed from the sample area of 8 m2 of each plot. Aliquots of 1 kg per plot were collected from each plot sample area, identified, weighed and fractionated according to the morphological components (leaf blades, stems and dead material) of elephant grass varieties and butterfly pea (leaves, stems and pods) separately. Thereafter, this material was dried in a forced-air oven at 55°C or until constant weight. Biomass accumulations of leaves and stems (elephant grass varieties + butterfly pea) were measured per harvest and per year, as well as the biomass accumulations of senescent material of grasses plus the pods of the legume. These productive aspects were estimated based on the DM content (AOAC DM, 930.15), determined according to the AOAC (Horwitz, Reference Horwitz2005).
Herbage bulk density was estimated using the ratio between herbage accumulation per harvest and average plants' height, estimated in four points of the sample area (8 m2). The number of leaves per tiller of elephant grass varieties and the number of leaves per stem of butterfly pea were estimated in four plants sampled from each plot. Four stems per plant were measured to count the butterfly pea leaves. Also, the average stem length of elephant grass was measured with the aid of a ruler graduated in cm, in four plants within each plot, as well as the average plants' height.
Light interception, LAI, light extinction coefficient (k) and RUE were obtained using information collected from the AccuPAR LP-80 canopy analyzer. Six readings above and below the canopies of each plot were performed in each evaluation. The device was transversally positioned regarding the rows of elephant grass varieties and butterfly pea, and the readings were performed at approximately 12.00 p.m., GMT in Brasilia, Brazil, under incident radiation according to the manufacturer's recommendation (Meter Group, 2018). The coefficient of light extinction was calculated using the equation proposed by Sheehy and Cooper (Reference Sheehy and Cooper1973), based on the Beer–Lambert law, assuming that the leaves were randomly distributed in the canopy:

where k is the light extinction coefficient, PAR is the photosynthetically active radiation and LAI is the leaf area index.
The RUE (g DM/MJ) was calculated by the ratio between the herbage accumulation rate estimated in g DM/m2 per day, and the unit of intercepted PAR (PARi) estimated in MJ/m2 per day. The PARi was determined by the difference between the PAR above and below the canopies converted from μmol/m2 per s to MJ/m2 per day (Ahmed and Ahmad, Reference Ahmed and Ahmad2020; Reis and Ribeiro, Reference Reis and Ribeiro2020). The RUE is often determined by a slope inclination of the regression between herbage accumulation and the intercepted PAR, but the estimation by the direct ratio is more accurate, considering that the slope inclination is equivalent to the RUE only when the intercept is zero (Druille et al., Reference Druille, Oyarzabal and Oesterheld2019). Furthermore, the non-fibre carbohydrate (NFC) content was calculated according to Hall (Reference Hall2003), using the below equation:

where NFC is the non-fibre carbohydrate content; NDFap is the content of neutral detergent fibre free of ashes (AOAC 942.05) and proteins; CP (AOAC 954.01) is the crude protein content and EE (AOAC 920.39) is the ether extract. These chemical analyses were realized according to the AOAC methodologies (Horwitz, Reference Horwitz2005).
Data were subjected to residual normality test (residues × quantiles) and analysed with the aid of PROC MIXED from SAS® OnDemand for Academics (SAS, 2014). Elephant grass variety, cropping system, year and their interactions were fixed effects. The effects of harvest, blocks and their interactions were random ones. When the F-test was significant (P < 0.05), the means were compared by the probability of difference (‘pdiff’) adjusted by Tukey's test (P < 0.05). The statistical model was:

where Y ijklm is the dependent variable; μ is the overall mean; αi is the fixed effect of elephant grass variety (1–4); βj is the fixed effect of cropping system (1–2); γ k is the fixed effect of the year (1–2); (αβ)ij is the interaction effect between elephant grass variety and cropping system; (αγ)ik is the interaction effect between elephant grass variety and year; (βγ)jk is the interaction effect between cropping system and year; (αβγ)ijk is the triple interaction between fixed effects; ω l is the random effect of the block (1–4); δ m is the random effect of harvest per year (1–4) and eijklm is the residual error of each observation.
Results
There were interaction effects between elephant grass variety and cropping system on biomass accumulations of leaves (Table 1) and stems (Table 2) per harvest and per year, besides on the varieties' senescent material (Table 3). Greater leaf biomass accumulation per year was observed for the dwarf Mott variety and the tall-type elephant B in the monocropping system while lower leaf accumulations per harvesting and year were found in the ‘Taiwan A-146 2.37 + butterfly pea’ treatment. Furthermore, there was an interaction effect between the evaluation year and cropping system on the leaf biomass accumulation. Greater values were observed in year 1 under the monocropping system compared to year 2, contrasting the intercrop system (Table 1), in which the leaf biomass accumulations displayed no significant differences.
Table 1. Leaf biomass accumulation averaged over harvest times and years of elephant grass varieties (C. purpureus Schum.) under monocropping or intercropped with the butterfly pea (C. ternatea L.)
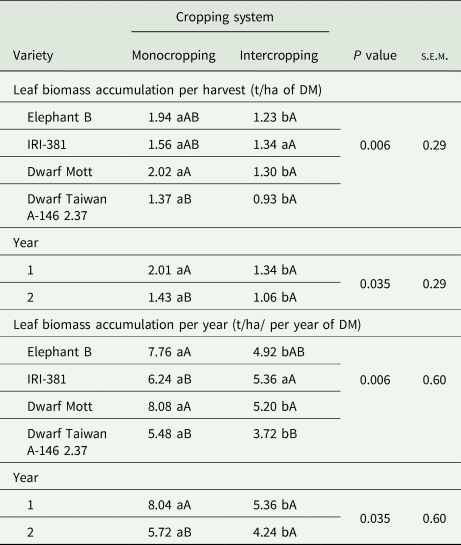
s.e.m., standard error of mean; DM, dry matter.
Leaf biomass accumulation was based on DM content. Year 1, 2019; year 2, 2020.
Means followed by the same lowercase letters within a row, and the same uppercase letter within a column for the same variable, do not differ from each other by the Tukey's test (P < 0.05).
Table 2. Stem biomass accumulation averaged over harvest times and years of elephant grass varieties (C. purpureus Schum.) under monocropping or intercropped with the butterfly pea (C. ternatea L.)
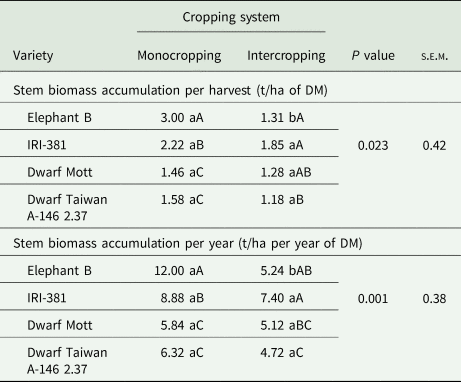
s.e.m., standard error of mean; DM, dry matter.
Leaf biomass accumulation was based on DM content.
Means followed by the same lowercase letters within a row, and the same uppercase letter within a column for the same variable, do not differ from each other by the Tukey's test (P < 0.05).
Table 3. Senescent biomass accumulation averaged over harvest times and years of elephant grass varieties (C. purpureus Schum.) under monocropping or intercropped with the butterfly pea (C. ternatea L.)

s.e.m., standard error of mean; DM, dry matter.
Leaf biomass accumulation was based on DM content.
Means followed by same lowercase letters within a row, and same uppercase letter within a column for a same variable, do not differ from each other by the Tukey's test (P < 0.05).
In the elephant grass monocrops, the tall varieties provided greater stem biomass accumulation (Table 2) while more senescent material was harvested in the dwarf Mott variety (Table 3). Also, there were isolated effects of evaluation year on the biomass accumulations of stems per harvest and year (P < 0.001), but without interactions. The accumulations of stems were 2.32 t/ha per harvest and 9.84 t/ha per year in year 1, contrasting the results found in year 2, which were 1.28 t/ha per harvest and 5.12 t/ha per year.
The presence of the elephant grass varieties did not affect the pod biomass accumulation of butterfly pea (P = 0.878) per harvest or per year, which averaged 0.19 t/ha per harvest and 0.76 t/ha per year. No year effect was observed too. There was an interaction effect of the cropping system and elephant grass variety on the herbage bulk density. Greater values were found in the canopies of dwarf Mott than in the canopies of most other treatments (Table 4). There were variety effects on the stem length and number of leaves per tiller of elephant grass. A greater number of leaves plus shorter stems were observed in the dwarf Mott variety than in the others (Table 5). The elephant grass varieties also differed significantly for the light interception and LAI (Table 5). Canopies of the dwarf Mott stood out with the greatest light interception and LAI. Regarding the number of leaves per stem in butterfly pea, a significant difference was observed between years (P = 0.001), with a higher value of 9 observed in year 1 compared to 7 in year 2. The elephant grass varieties had no significant effect on the intercrop butterfly pea for the number of leaves per stem (P = 0.443).
Table 4. Herbage bulk density (kg/ha per cm) of canopies of elephant grass populations (C. purpureus Schum.) averaged over harvest times and years under monocropping or intercropped with butterfly pea (C. ternatea L.)

s.e.m., standard error of mean.
Means followed by the same lowercase letters within a row, and the same uppercase letter within a column do not differ from each other by the Tukey's test (P < 0.05).
Table 5. Stem length (SL), number of leaves per tiller (NLT), light interception (LI) and LAI of elephant grass varieties (C. purpureus Schum.) averaged over harvest times and years

s.e.m., standard error of mean.
Means followed by the same uppercase letter within a column for the same variable, do not differ from each other by the Tukey's test (P < 0.05).
Furthermore, an interaction effect between variety and cropping system was found on the light extinction coefficient (k). Greater k values were observed in the dwarf Mott canopies under monocropping compared to its respective intercropping with the legume butterfly pea, while other varieties showed consistent k values across cropping systems (Table 6). Nevertheless, no differences in k values were found between the varieties within the intercropping system.
Table 6. Light extinction coefficient (k) of canopies from elephant grass varieties (C. purpureus Schum.) averaged over harvest times and years under monocropping or intercropped with butterfly pea (C. ternatea L.)

s.e.m., standard error of mean.
Means followed by the same lowercase letters within a row, and the same uppercase letter within a column do not differ from each other by the Tukey's test (P < 0.05).
There was an interaction effect between variety and year on the RUE (Table 7). In year 1, the elephant B canopies displayed greater RUE than those formed by the dwarf varieties Mott and Taiwan A-146 2.37, but with no differences regarded to the canopies composed of IRI-381. In addition, the RUEs were statistically on par for all the varieties in year 2. However, the RUEs of the tall varieties were greater in the first evaluation year than in the second one.
Table 7. RUE (g DM/MJ) of canopies from elephant grass varieties (C. purpureus Schum.) under monocropping or intercropped with butterfly pea (C. ternatea L.) averaged over harvest times in years 1 and 2

s.e.m., standard error of mean; year 1, 2019; year 2, 2020.
Means followed by the same lowercase letters within a row, and the same uppercase letter within a column, do not differ from each other by the Tukey's test (P < 0.05).
There were interaction effects between variety and year on the NFC contents and between variety and cropping system for the same variable. The herbage harvested from dwarf Mott had greater content of NFC than the other varieties, regardless of the evaluation year (Table 8). Also, greater NFC contents were found in year 1 compared to year 2 for all varieties evaluated. The intercropping with the butterfly pea provided greater NFC contents compared to the elephant grass under monocropping, at least in Taiwan A-146 2.37, elephant B and IRI-381 varieties.
Table 8. NFC (g/kg DM) content of elephant grass varieties (C. purpureus Schum.) under monocropping or intercropped with butterfly pea (C. ternatea L.) averaged over harvest times in years 1 and 2

s.e.m., standard error of mean; year 1, 2019; year 2, 2020.
Means followed by the same lowercase letter within a row, and the same uppercase letter within a column for the same variable, do not differ from each other by the Tukey's test (P < 0.05).
Discussion
Results of light interception, LAI and RUE were low when considering the potential of leaf biomass accumulation of elephant grass varieties and butterfly pea (Woodard et al., Reference Woodard, Prine and Bachrein1993; Viana et al., Reference Viana, Mello, Lira, Guim, Dubeux Junior, Santos and Cunha2015; Druille et al., Reference Druille, Oyarzabal and Oesterheld2019; Oguis et al., Reference Oguis, Gilding, Jackson and Craik2019). Adverse weather conditions in year 2 related to low rainfall, high temperatures (Fig. 1) and evapotranspiration, added to the ageing of the plant stands, likely contributed to smaller leaf sizes, less tillering/branching, leaf area, the water content in tissues and plant vigour, leading to less leaf biomass accumulation and NFC contents observed in that year. Plants under drought stress often reduce or cease their photosynthetic activities and the production of photoassimilates, resulting in reduced growth rates, light use efficiency and herbage accumulation (Jajoo and Mathur, Reference Jajoo and Mathur2021; Seleiman et al., Reference Seleiman, Al-Suhaibani, Ali, Akmal, Alotaibi, Refay and Battaglia2021).
However, not only the weather conditions influenced the results. The cut management adopted in this study probably affected the LAI and RUE, plus the canopy structure and leaf biomass accumulation, because the dynamic of vegetation in cut-and-carry systems is very different from that of pastures. The herbage harvest in cut-and-carry systems occurs under strong intensities, often below 5 cm of stubble height, with the removal of almost all the photosynthetic apparatus (leaves), modifying the leaf area of the canopies (Cunha et al., Reference Cunha, Lira, Santos, Freitas, Dubeux Junior, Mello and Martins2011; Souza et al., Reference Souza, Santos, Cunha, Gonçalves, Silva, Mello and Dubeux2021). In addition, the elephant grass was spaced 1.0 m apart, which probably altered the light interception and the canopy structure. Wide spacing between rows may reduce the soil cover by vegetation, consequently reducing light interception, especially in elephant grass canopies composed of clumps that naturally do not cover the land as stoloniferous grasses do (Silva et al., Reference Silva, Sales, Lemos, Silva, Ribeiro, Santos, Mello and Cunha2021a).
The tall variety elephant B and the dwarf-type Mott, despite their differences in stature, stood out with greater annual leaf accumulations. Canopies formed by dwarf Mott intercropped or not with the butterfly pea, presented greater herbage bulk density, light interception and LAI because of their greater leaf productivity, the substantially higher number of leaves per tiller and short stems (Viana et al., Reference Viana, Mello, Guim, Lira, Dubeux Júnior, Santos and Cunha2018; Silva et al., Reference Silva, Sales, Lemos, Silva, Ribeiro, Santos, Mello and Cunha2021a). Dwarf elephant grass cultivars like Mott and BRS Kurumi were selected to be exploited under grazing management, and they have such characteristics as a great leaf–stem ratio and short internodes (Sollenberger et al., Reference Sollenberger, Prine, Ocumpaugh, Hanna, Jones Junior, Schank and Kalmbacher1989; Pereira et al., Reference Pereira, Lédo and Machado2017; Silva et al., Reference Silva, Sales, Lemos, Silva, Ribeiro, Santos, Mello and Cunha2021a), which favour greater leaf biomass accumulation and the ability to form dense canopies. In this context, Gomide et al. (Reference Gomide, Chaves, Ribeiro, Sollenberger, Paciullo, Pereira and Morenz2015) also highlighted a significant herbage bulk density in swards composed of the dwarf BRS Kurumi while Viana et al. (Reference Viana, Mello, Lira, Guim, Dubeux Junior, Santos and Cunha2015) reported a notable number of leaves per tiller in the dwarf Mott. Furthermore, Cunha et al. (Reference Cunha, Lira, Santos, Freitas, Dubeux Junior, Mello and Martins2011) reported greater LAI in the dwarf Mott variety compared to the tall types elephant B and IRI-381.
Contrastingly, the dwarf variety Taiwan A-146 2.37 exhibited the lowest leaf accumulation per year, in both cropping systems. This variety and other ‘Taiwan A-146’ progenies display narrower and erect leaves, besides thin stems and moderate-to-short size (100 cm) (Cheng, Reference Cheng1991; Viana et al., Reference Viana, Mello, Guim, Lira, Dubeux Júnior, Santos and Cunha2018), resulting in lower herbage accumulation than other varieties such as elephant B, IRI-381 and dwarf Mott (Silva et al., Reference Silva, Santos, Dubeux, Lira, Ferreira, Freitas and Silva2010; Cunha et al., Reference Cunha, Lira, Santos, Freitas, Dubeux Junior, Mello and Martins2011; Viana et al., Reference Viana, Mello, Lira, Guim, Dubeux Junior, Santos and Cunha2015).
The differences in biomass accumulations of leaves and stems, besides the contrasting herbage bulk density between the cropping systems, observed in almost all the studied treatments, likely occurred under the influence of nitrogen fertilization with inorganic fertilizers carried out only in the monocropping system. Under the intercropped treatments, the N fixed through butterfly pea inclusion might not be enough to meet the elephant grass demands and provide competitive N nutrition compared to the fertilized monocrop. Warm-season grasses, such as elephant grass, display efficient responses to nitrogen fertilization and availability (Silva et al., Reference Silva, Carvalho, Morenz, Silva, Santos and Santos2018). The leaves of higher plants act like efficient drains of soil resources, directly or indirectly (Taiz et al., Reference Taiz, Zeiger, Moller and Murphy2017). Thus, their mineral nutrition using elevated doses of nitrogen, and quick-soluble fertilizers like urea, tends to increase leaf production (Marschner, Reference Marschner2011).
Nevertheless, previous studies have shown the beneficial effects of intercropping forage grasses with legumes on both herbage and leaf biomass accumulation. Oliveira et al. (Reference Oliveira, Demeu, Bonatti, Torres and Teodoro2017) did not observe differences in herbage accumulation per harvest of the elephant grass under monocropping (7.80 t/ha), or intercropped (8.48 t/ha) with leucena [Leucaena leucocephala (Lam.) de Wit.], in which they had applied supplemental N under both cropping systems. Vieira et al. (Reference Vieira, Olivo, Adams, Sauthier, Proença, Oliveira, Santos, Schiafino, Tonin, Godoy, Arrial and Casagrande2019) observed a greater percentage of leaf blades in elephant grass (44.6%) intercropped with forage peanut (Arachis pintoi Krap. and Greg.) under grazing management, compared to those from the elephant grass under monocropping (38.5%). The crops had received nitrogen fertilizers in both cropping systems. These results were favourable for the intercropping systems compared to those recorded in our trial. However, we recorded numerous adversities related to ecological factors that probably influenced the plants' growth and development, particularly the atypical climate conditions of the study site in year 2 characterized by lower precipitation, high temperatures and evapotranspiration, contrasting sharply with the weather conditions of year 1 (Fig. 1). Additionally, as mentioned before, the intercropping system had no supplemental N applied.
In year 1, the tall variety elephant B showed greater RUE compared to the dwarf varieties Mott and Taiwan A-146 2.37. Since this variable represents a ratio between herbage accumulation and intercepted photosynthetic active radiation (Druille et al., Reference Druille, Oyarzabal and Oesterheld2019), a lower leaf–stem ratio can justify the better efficiency of the tall varieties in cut-and-carry systems.
When managed under a greater harvesting intensity, elephant grass varieties have basilar tillers in the majority, and the stems of tall varieties are long, heavy, more lignified, and have greater DM content (Paciullo et al., Reference Paciullo, Deresz, Aroeira, Morenz and Verneque2003; Silva et al., Reference Silva, Santos, Dubeux, Lira, Ferreira, Freitas and Silva2010, Reference Silva, Cunha, Santos, Magalhães, Mello, Silva and Souza2021b). This became evident as the tall varieties provided such higher biomass stem accumulation than the dwarf types, especially in the monocropping system. The differences in stem lengths were notable too. The stem biomass accumulation in tall elephant grass varieties contributes to greater herbage accumulation, despite diminishing the nutritive value, especially in cut-and-carry systems (Souza et al., Reference Souza, Santos, Cunha, Gonçalves, Silva, Mello and Dubeux2021). Conversely, the dwarf varieties display genetic traits that cease their stem elongation earlier (Yan et al., Reference Yan, Li, Lu, Gao, Lai, Yao and Zhang2021), reducing the herbage production in comparison with tall varieties, but these same characteristics tend to improve their nutritive value (Pereira et al., Reference Pereira, Lédo and Machado2017; Silva et al., Reference Silva, Cunha, Santos, Magalhães, Mello, Silva and Souza2021b). These aspects could be observed in the dwarf Mott, especially the very short stems.
The lower RUE observed in the dwarf varieties could have various reasons. Concerning the dwarf Mott, the combination of greater leaf biomass accumulation, greater light interception and greater light extinction led to a low efficiency to use photosynthetic radiation. In this scenario, there was a low degree of light penetration to the base of the canopy of the dwarf Mott, causing auto-shading that resulted in high percentages of dead material among lower leaves, due to the fast senescence of shaded leaves in basilar strata (Gastal and Lemaire, Reference Gastal and Lemaire2015; Geremia et al., Reference Geremia, Crestani, Mascheroni, Carnevalli, Mourão and Da Silva2018). In this case, the long harvest interval of 60 days likely contributed to the great senescent biomass accumulation. Therefore, increasing harvest frequencies with shorter intervals for this cultivar compared to the tall ones may alleviate auto-shading and senescence issues, while enhancing herbage accumulation and quality (Da Silva et al., Reference Da Silva, Sbrissia and Pereira2015). Concerning the dwarf Taiwan A-146 2.37, the low RUE values were associated with the low light interception in its canopies, possibly because of its morphological traits like thin stems, and narrow and upright leaves (Cheng, Reference Cheng1991).
Although the intercrop systems resulted in lower accumulations of leaves and stems than the monocrops, no significant differences were observed in the comparisons of intercrops and monocrops for RUE results. The positive effect of the butterfly pea inclusion in the system likely matched the positive effect of N fertilization using urea to increase plants' light use efficiency (Silva et al., Reference Silva, Carvalho, Morenz, Silva, Santos and Santos2018), through nitrogen inputs by litter deposition, root decomposition or even by direct transfer (Schultze-Kraft et al., Reference Schultze-Kraft, Rao, Peters, Clements, Bai and Liu2018). Furthermore, the growth habits of both elephant grass (erect) and butterfly pea (a climbing legume) could have contributed to a greater RUE by plants in the intercrops, alleviating the interspecific competition for the photosynthetic active radiation (Boddey et al., Reference Boddey, Casagrande, Homem and Alves2020).
Despite low RUE, the dwarf-type elephant grass Mott displayed greater NFC, as well as the intercrops compared to the monocrops. Herbaceous legumes like butterfly pea often have greater NFC contents than warm-season grasses (Lee, Reference Lee2018), which could explain the elevated NFC concentration in the intercrops. Moreover, the NFC results allowed an understanding of how the dwarf Mott variety was able to maintain carbohydrate reserves (e.g. non-structural carbohydrates such as starch and saccharose) while limiting herbage accumulation by reducing stem elongations. In cut-and-carry systems, the organic reserves are more efficient than the residual leaf area to ensure better plant regrowth capacity, crop persistence and quicker canopy reestablishment. These carbohydrate reserves are located mainly in the base of the stems, but also in rhizomes and roots (Silva et al., Reference Silva, Sales, Lemos, Silva, Ribeiro, Santos, Mello and Cunha2021a). Dwarf Mott variety tends to form dense clumps with large crowns and thick stems, which are less lignified than those of tall elephant grass varieties (Sollenberger et al., Reference Sollenberger, Prine, Ocumpaugh, Hanna, Jones Junior, Schank and Kalmbacher1989; Viana et al., Reference Viana, Mello, Guim, Lira, Dubeux Júnior, Santos and Cunha2018; Souza et al., Reference Souza, Santos, Cunha, Gonçalves, Silva, Mello and Dubeux2021). These morphophysiological characteristics contributed to greater herbage bulk density of the dwarf Mott canopies than those of other varieties, even with the elevated accumulation of senescent biomass and low RUE, probably caused by the long harvest interval of 60 days.
Conclusions
Tall varieties elephant B and IRI-381 displayed greater efficiency in intercepting the radiation to accumulate herbage via stem accumulation in a 60-day interval, but this characteristic tends to reduce the NFCs in the vegetal tissue. Tall varieties of elephant grass can be harvested at 60-day frequency with no losses in RUE and maintaining suitable herbage accumulation.
Dwarf Mott variety exhibited short stems and great leaf biomass accumulation that favoured denser canopies and higher content of NFCs in vegetal tissue. It is speculated that dwarf varieties may display comparable or better RUE under shorter harvest intervals (e.g. 45 days), which can be evaluated in further studies.
The butterfly pea's inclusion as a climber legume intercrop in elephant grass cut-and-carry systems did not impair the penetration of photosynthetic radiation to the canopy base, despite its climbing nature. Planting butterfly pea legume into rows of elephant grass varieties can be adopted with no significant losses in RUE caused by light extinction, regardless of the grass stature.
Acknowledgements
The authors would like to acknowledge all the employees and contributors from the Sugarcane Experimental Station of Carpina for their assistance to carry out this study.
Author contributions
P. H. F. Silva, M. V. F. Santos, A. C. L. Mello and M. V. Cunha conceived and designed the study. P. H. F. Silva, T. B. Sales, E. R. Costa, L. M. P. Guimarães and D. E. Simões Neto conducted data gathering. P. H. F. Silva and M. V. Cunha performed statistical analyses. P. H. F. Silva, J. J. Coêlho and M. V. Cunha wrote the article.
Financial support
This study was financed in part by the Coordenação de Aperfeiçoamento de Pessoal de Nível Superior – Brasil (CAPES) – Finance Code 001, and in part by the Conselho Nacional de Desenvolvimento Científico e Tecnológico (CNPq).
Conflict of interest
The authors declare no conflict of interest.