1. Introduction
Ice sheets and glaciers currently cover 12.5% of Earth's land surface (Bamber and others, Reference Bamber, Westaway, Marzeion and Wouters2018). At present, ice masses consist of the continental ice sheets in Antarctica and Greenland and more than 200 000 glaciers distinct from the ice sheets (Pfeffer and others, Reference Pfeffer2014), representing potential sea-level rise of 57.9 ± 0.9 m (Morlighem and others, Reference Morlighem2020), 7.4 ± 0.05 m (Morlighem and others, Reference Morlighem2017) and <0.5 m (Farinotti and others, Reference Farinotti2019), respectively. Together, these ice bodies store 69% of the world's fresh water (Gleick, Reference Gleick and Schneider1996).
Glaciers form where snow accumulation exceeds the annual melt over decades to centuries. As glaciers form where climatic conditions permit (i.e. cold regions with sufficient precipitation), they often straddle complex mountainous terrain where different sections of the ice surface drain into different valleys. In addition, depending on the topographic as well as present and past climatic setting, glacier complexes can build ice caps or icefields that drain ice from common accumulation zones via outlet glaciers to different watersheds (Table 1). Traditionally, and for hydrological reasons, glacier complexes have been divided into glaciers, often with distinct names, based on their drainage basins.
Table 1. Terminology related to glaciers and glacier complexes according to Cogley and others (Reference Cogley2011)

a Objects that may be divisible into more than one glacier (e.g. ice caps and icefields).
As a consequence, the size of an ice body (and total number of glaciers) will depend on whether or not a glacier complex (e.g. ice caps and icefields) is considered as one single entity or subdivided into glaciers based on drainage divides. These can be calculated from a digital elevation model (DEM) representing the glacier surface and watershed algorithms that analyze the direction of surface flow with respect to a pour point outside the glacier extent (e.g. Bolch and others, Reference Bolch, Menounos and Wheate2010). While the division is often straightforward in regions where glaciers are surrounded by steep topography (Fig. 1a), it is much more difficult for glacier complexes that cover much or all of the underlying topography, and hence make identifying individual flow basins less obvious. This is especially true of ice caps with their radial flow, as they may initially be classified as one entity rather than a collection of glaciers having formed an ice cap (Fig. 1b). For icefields, it is challenging to define the correct position of ice divides as their outlet glaciers originate in relatively flat surface topography (Figs 1c, d). When a DEM of limited quality is used to derive the ice divides, the somehow arbitrary glacier separations visible in Fig. 1c can result. Separating glacier complexes into glaciers is further challenged by climate-change-driven shifts in ice divides and separation of glacier tongues and, in practice, by the lack of high-quality, contemporaneous, high-resolution elevation data.

Fig. 1. Examples of glaciers and glacier complexes: (a) Aletsch Glacier in Switzerland, (b) Vatnajökull Ice Cap in Iceland and (c) Northern Ellesmere Icefield in the Canadian Arctic. Subplot (d) shows the Holtedalfonna-Isachsenfonna Icefields in Svalbard with a zoom to its contiguous outlet glaciers. Outlines of the glaciers and of glacier complexes are shown in blue and red, respectively; the background image is the ESRI World Imagery base map (ESRI, 2022).
The appropriate choice of glacier versus glacier complex varies with the purpose of the study and the perspective of the user. For example, considering glacier complexes as a whole might be sufficient to map glaciers for land-cover classifications or for projects where ice bodies represent a natural barrier for land-based infrastructure. Conversely, dividing a glacier complex into glaciers is important to properly evaluate the meltwater input to an individual hydrological drainage basin or to determine the mass flux to marine-terminating glacier fronts or ice shelves. Further, whether to divide a glacier complex for modeling purposes depends on the model type. While some models rely on a glacier inventory that includes data for each glacier draining a glacier complex (e.g. Huss and Hock, Reference Huss and Hock2015; Maussion and others, Reference Maussion2019), other models treat the ice cover as a whole and do not require individual glacier inputs (e.g. Immerzeel and others, Reference Immerzeel, van Beek, Konz, Shrestha and Bierkens2012; Seibert and others, Reference Seibert, Vis, Kohn, Weiler and Stahl2018).
Despite more than 125 years of internationally coordinated glacier monitoring (Zemp and others, Reference Zemp, Kargel, Leonard, Bishop, Kääb and Raup2014; Allison and others, Reference Allison2019) that have resulted in, among others, several glacier inventories (WGMS, 1989; Raup and others, Reference Raup2007; Pfeffer and others, Reference Pfeffer2014), no official list has been compiled of the world's largest glaciers, although numerous inconsistent rankings exist in popular literature. This may in part be due to the aforementioned topographic complexity of glacier surfaces which complicates unambiguous drawing of glacier boundaries, further compounded by the fact that existing glacier inventories have not been designed for this purpose.
Here, we present the first systematic assessment of the largest glaciers in the world, based on area, over two domains: the seven geographical continents and the 19 first-order glacier regions as defined by the Global Terrestrial Network for Glaciers (GTN-G, 2017). Given the considerations above, we provide two rankings – one for glacier complexes and one for glaciers – using the Global Land Ice Measurements from Space (GLIMS) glacier data and the Randolph Glacier Inventory (RGI) 6.0. In addition, we discuss the main challenges for such rankings and provide an outlook on future work.
2. Glacier monitoring and inventories
Internationally coordinated glacier monitoring was initiated as early as 1894 (Forel, Reference Forel1895; Allison and others, Reference Allison2019), with a main focus on compiling standardized observations of changes in glacier length, and later, changes in glacier volume and mass (Haeberli, Reference Haeberli, Orlove, Wiegandt and Luckman2008). The need for a worldwide inventory of existing ‘perennial ice and snow masses’ was first considered during the International Hydrological Decade (IHD, 1965–74) and resulted in the World Glacier Inventory (WGMS, 1989) including statistical information, mainly based on aerial photographs and maps, of about one-third of the global glacier area. In 2005, the World Glacier Inventory was complemented, and later superseded, by the GLIMS glacier database, which was designed to store multi-temporal digital vector outlines of the world's glaciers and related topographic information primarily derived from optical satellite images (GLIMS Consortium, 2005; Paul and others, Reference Paul2009). By 2013, GLIMS covered ~58% of global glacierized area including multi-temporal coverage for thousands of glaciers. The version of GLIMS used for this analysis contains ~383 000 glacier outlines including multiple outlines from different dates for many glaciers.
Stimulated by the Fifth Assessment Report of the Intergovernmental Panel on Climate Change (IPCC, 2013) and an increasing need for complete global coverage for large-scale glaciological applications, a nearly complete global inventory with one vector outline for each glacier outside the ice sheets was produced. Named the Randolph Glacier Inventory (RGI), the first version (Pfeffer and others, Reference Pfeffer2014) complemented glacier outlines from GLIMS with new datasets from other sources originating mostly from the first decade of this century. The RGI has been updated several times since. The latest version (RGI 6.0; RGI Consortium, 2017) provides a snapshot for the beginning of the 21st century and includes ~215 000 glaciers covering an area of ~706 000 km2. Note that RGI 6.0 does not contain the glaciers on the Antarctic Peninsula while GLIMS does include them.
As a result of the historic development and data provision by individual analysts, the RGI and GLIMS databases are not fully consistent due to differences in how analysts divide glacier complexes. Such divisions are in many cases difficult to apply, be it for technical reasons (low-quality DEM) or methodological ones (e.g. circular ice caps, ice aprons, glaciers with interrupted profiles). The available inventories thus present a mixture of divided and undivided glacier complexes as well as glacier extents following different definitions. Indeed, this results in problems when trying to answer the question about the largest glaciers in the world. This is discussed further in the discussion section on Data consistency.
3. Methods
Glacier area (in km2) is used as an obvious measure of glacier size that can be extracted as attributes from both the GLIMS and RGI databases. It is the primary variable associated with glacier vector outlines, which can serve as a baseline input for ranking according to secondary variables such as glacier length, volume or mass. However, a system for ranking glaciers by size cannot be directly derived as noted in Section 2. In addition, some RGI outlines are older than GLIMS outlines. Therefore, we used both the GLIMS glacier database (version 20190304; GLIMS Consortium, 2005) and the RGI inventory (version 6.0; RGI Consortium, 2017) to compile one recent outline for each glacier in the world. Further, how an analyst defines a glacier and a glacier complex is important to the outcome of the analysis. Depending on the research question, these can be defined in different ways, which could lead to different results. Here, we use the definitions from Cogley and others (Reference Cogley2011) as listed in Table 1 when referring to glaciers, glacier complexes, ice caps or icefields.
Note that we do not consider the Greenland and Antarctic ice sheets in this analysis. However, we do include all glaciers peripheral to the Greenland Ice Sheet with connectivity levels 0 and 1 in RGI 6.0 (Rastner and others, Reference Rastner2012), which represent that the glacier is not connected to (i.e. physically separate from) the ice sheet or weakly connected (i.e. only touching the ice sheet at a distinct ice divide in the accumulation region, and not connected or only in contact in the ablation region), respectively. We also include all glaciers in the Antarctic periphery, which in RGI 6.0 consists of the glaciers on the Antarctic and Subantarctic Islands but lacks those on the Antarctic mainland.
We subdivided region 19 into the Antarctic mainland (second-order region 19-31 in GTN-G, 2017) and the Antarctic and Subantarctic Islands (second-order regions 19-01 to 19-24 in GTN-G, 2017). In addition, using GLIMS, we incorporate the ice body on the Antarctic Peninsula (north of 70° South) even though it is connected to the West Antarctic Ice Sheet and is, therefore, often considered part of the Antarctic Ice Sheet (Fretwell and others, Reference Fretwell2013; IMBIE Team, 2018; Seroussi and others, Reference Seroussi2020). Note that while other entities in Antarctica are also named glaciers (e.g. Thwaites and Pine Island), they are outlet glaciers of the Antarctic Ice Sheet (Fretwell and others, Reference Fretwell2013). As such, we have excluded them from this analysis.
We query both databases and extract the glacier area attribute from them for each of the seven geographical continents and the 19 first-order glacier regions (GTN-G, 2017) (Fig. 2) – excluding ice shelves and the two ice sheets as described above – and use the extracted quantities as the de facto value for glacier area. The results from these queries were reviewed, and the three largest glaciers per region were obtained. In cases where a glacier's size differed between GLIMS and RGI, we compared the dates of the measurements and chose the one with the more recent date. If the measurement dates matched (same year), the areas were averaged.

Fig. 2. Global overview of glacier area used in this analysis (blue) with the 19 first-order GTN-G glacier regions (GTN-G, 2017) (black outlines). Numbers refer to the GTN-G region numbers. Region 19 is subdivided into two regions (mainland and islands) but this subdivision is not shown on this map.
To determine the size of glacier complexes, they had to be identified first. Glaciers that shared common boundaries with one or more neighboring glaciers were merged at their common ice divides to create a glacier complex outline. Even when glaciers were only connected by a small glacier confluence, we chose not to change the original data and, hence, merge these into one glacier complex (see the discussion on Data consistency for more information). The area of each glacier complex was then determined using a Python planar area function in an equal-area projection. The GLIMS glacier database alone was used for this part of the analysis with two exceptions: the Greenland Periphery (region 5) and the Antarctic and Subantarctic Islands (regions 19-01 to 19-24) where RGI had to be used.
Glacier names are those given in the GLIMS and RGI datasets, when available. For glaciers without names in these datasets, we searched for a corresponding name in the Fluctuations of Glaciers database (WGMS, 2021), the World Glacier Inventory (WGMS and NSIDC, 2012), OpenStreetMap (2021) and in the scientific literature. If a name was still unknown, we named the glacier after a prominent geographic feature (e.g. Alexander Island Glacier No. 1). For glacier complexes, we used either an existing name (e.g. Southern Patagonian Icefield, Agassiz Ice Cap, Vatnajökull) – where available – or named the complex after its largest glacier(s) (e.g. Grosser Aletsch Glacier Complex, Gepatsch-Hintereis Glacier Complex), or a related prominent topographic feature (e.g. Vilcanota Glacier Complex, Western Kunlun Icefield). We recognize that some glaciers carried indigenous names before they were renamed by explorers. For example, Malaspina Glacier was Sít' Tlein (Thornton, Reference Thornton2012). For a list of the glaciers and glacier complexes for which we created the names, see Windnagel (Reference Windnagel2022).
4. Results
An overview of the ten largest glaciers and glacier complexes in the world is presented in Table 2, and the largest from each of the 19 GTN-G regions is shown in Figs 3 and 4. The three largest in the seven geographical continents are presented in Table 3. A complete list is given in Supplementary Table S1. The world's largest glacier complexes are located in the polar regions, and the ice body covering the Antarctic Peninsula (north of 70°S) is by far the largest, with an area of almost 81 000 km2. It consists of ~1500 ice bodies that are sometimes treated as glaciers (Huss and Farinotti, Reference Huss and Farinotti2014; Huber and others, Reference Huber, Cook, Paul and Zemp2017) and by others as part of the Antarctic Ice Sheet (Fretwell and others, Reference Fretwell2013). The second largest glacier complex covers an area of ~47 000 km2 and is located on Alexander Island in the Antarctic; this area excludes the ice shelves connected to the island. Third largest is the Malaspina-Seward Glacier Complex in Alaska covering 30 000 km2, followed by the Severny Island Northern Ice Cap in the Russian Arctic at just over 20 000 km2. Finally, in the Canadian Arctic, there is the Northern Ellesmere Icefield, the Prince of Wales Icefield and the Agassiz Ice Cap, with areas between 18 000 and 19 500 km2. Outside the polar regions, the Southern Patagonian Icefield is the largest glacier complex with a size of ~13 000 km2.

Fig. 3. Overview of the largest glacier complexes in each of the 19 first-order regions, sorted by area (largest to smallest). The region number is listed in the black box. The Region 19 subregions are listed as 19 M (Antarctic Mainland) and 19I (Antarctic and Subantarctic Islands). Glacier complexes are projected in local Universal Transverse Mercator, centered at the polygon's centroid, chosen as a best compromise to minimize distortion in shape and area. Areas are computed using an equal area projection. The area differences between these two coordinate reference systems are <0.1%. Note that we considered the ice body on the Antarctic Peninsula as an ice complex although it is connected to the Antarctic Ice Sheet.

Fig. 4. Overview of the largest glaciers in each of the 19 first-order regions, sorted by area (largest to smallest). Display methods are the same as Figure 3.
Table 2. The world's ten largest glacier complexes and glaciers, excluding the ice sheets but including glaciers in their periphery as well as the ice body on the Antarctic Peninsula
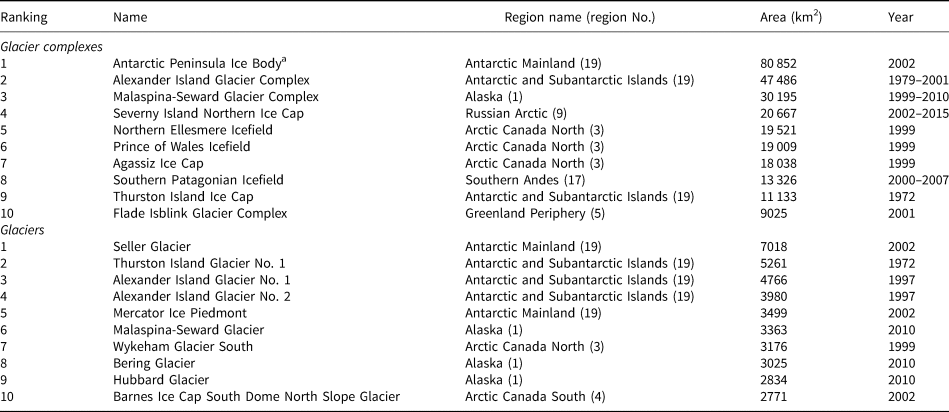
‘Year’ indicates the year or range of years the area refers to.
a Note that we consider the ice body on the Antarctic Peninsula as a glacier complex although it is connected to the Antarctic Ice Sheet.
Table 3. The three largest glacier complexes and glaciers for each of the seven geographical continents, excluding ice sheets but including glaciers in their periphery and the ice body on the Antarctic Peninsula

'Year' indicates the year or range of years the area refers to.
a Note that we considered the ice body on the Antarctic Peninsula as an ice complex although it is connected to the Antarctic Ice Sheet.
The world's largest (individual) glaciers are found in the Antarctic, led by Seller Glacier (7018 km2) on the Antarctic Peninsula, and then Thurston Island Glacier No. 1 (5261 km2) and Alexander Island Glacier No. 1 (4766 km2), both located on Antarctic islands. Outside the Antarctic, the largest glaciers (~3000 km2) are Malaspina-Seward Glacier in Alaska followed by Wykeham Glacier South in the Canadian Arctic and Bering Glacier in Alaska.
For context, the sum of the total area of the ten largest glaciers is ~5% of the total area of all the glaciers in the world, which is ~786 800 km2, arrived at by summing the total area from RGI with the total area of the glaciers on the Antarctic Peninsula. The total area of the ten largest glacier complexes is just over 34% of the total glacier area on Earth.
Note that the uncertainty in the outlines in these databases is an important point to consider when assessing the results from this analysis. GLIMS provides uncertainties for individual outlines (for some glaciers), but RGI does not. Hence, we can only discuss the potential influence of these uncertainties on glacier area and ranking but not provide quantitative error estimates for each of the largest glaciers (see the discussion on Data quality for further information).
5. Discussion
5.1 The largest glaciers and their geographical occurrence
Our study shows that the question of which glaciers are largest depends on whether, and how, glacier complexes are distinguished from individual glaciers. We find that the largest glacier complexes cover areas larger than 10 000 km2, whereas the largest glaciers cover up to 10 000 km2 (Table 2). For comparison, the largest glacier complexes cover areas similar to smaller countries (e.g. Bhutan or Austria), smaller US states (e.g. New Jersey or South Carolina) or some islands (e.g. Hokkaido (the 21st largest island in the world) or Tasmania), and yet are still orders of magnitudes smaller than the Greenland and Antarctic Ice Sheets, encompassing 1.7 × 106 km2 (Zwally and others, Reference Zwally, Giovinetto, Beckley and Saba2012) and 12.3 × 106 km2 (Zwally and others, Reference Zwally, Giovinetto, Beckley and Saba2012; Fretwell and others, Reference Fretwell2013), respectively.
Glacier size is a function of both climate and underlying topography. In the present study, we show that the largest glaciers are found in the polar regions, where low temperatures and relatively flat topographies have allowed large icefields and ice caps to grow and build major glacier complexes – over long time periods – in spite of low annual precipitation (Braithwaite and Hughes, Reference Braithwaite and Hughes2020). Outside the Arctic and Antarctic regions, the largest glacier complexes occur in Alaska, Patagonia and Iceland where glaciers profit from the high annual precipitation of maritime climate regimes (Braithwaite and Hughes, Reference Braithwaite and Hughes2020). In High Mountain Asia, steep topography results in well-defined catchments but limits the area of glaciers and the formation of very large glacier complexes even though some of the longest glaciers in the world exist in this region (Machguth and Huss, Reference Machguth and Huss2014).
5.2 Challenges related to ranking glaciers by area
Which glaciers are the largest in the world? What seems to be a simple question, in fact, is difficult to answer. Not only does one need a clear definition of what a glacier and glacier complex are, but also a globally complete dataset with a consistency that allows for a related comparison. In the following sections, we elaborate on the key challenges to ranking glaciers by area.
5.2.1 Definition
Glaciers form different glacier types depending on topographic and climatic conditions. These conditions differ from region to region and across continents. Consequently, a generally accepted definition is the most fundamental requirement to rank the world's glaciers by area. The ‘Glossary of glacier mass-balance and related terms’ by Cogley and others (Reference Cogley2011) provides consensus definitions (Table 1). In particular, the differentiation between glaciers and glacier complexes emerged as essential. While this differentiation is theoretically reasonable, the related practical implementation comes with some complications. Separating individual glaciers – such as Grosser Aletsch Glacier in the European Alps (Fig. 1a) – is straightforward in regions of steep high-mountain topography with clearly defined hydrological basins. However, the task is more challenging when several glaciers form complexes in regions where the topography is flat, as illustrated by Kronebreen, Infantfonna, Kongsvegen and Sidevegen, which are neighboring glaciers in Svalbard that are calving into Kongsfjord and share a common glacier tongue (Fig. 1d).
In this case, the division might be justified as the individual tongues exhibit different flow dynamics, but other glaciers that merge from individual basins into a common tongue or only touch a trunk glacier as a tributary without contributing to its flow remain undivided. This inconsistency is difficult to solve and might also vary over time, necessitating a time stamp for the outline when size rankings are performed (see Tables 2 and 3).
The complex nature of glacier topography is well demonstrated by the Northern Ellesmere Icefield (Arctic Canada North) where hundreds of glaciers cover the mountainous terrain but are separated by mountain topography in their ablation regions. These glaciers partly share ambiguous ice divides (that change with the DEMs used to calculate them) and have tongues that are fed from accumulation zones in opposite valleys, connecting two icefields to form an even larger glacier complex (Fig. 1c). In addition, we note that glacier names – if available in the global datasets – often originate from regional or national contexts and, hence, are not always consistent with the classification based on topography. For example, the term for ‘glacier’ in Iceland is ‘jökull’, but it is used for both an ice cap and its outlet glaciers (Sigurdsson and Williams, Reference Sigurdsson and Williams2008). The same is true in Norway for the word ‘breen’ (Andreassen and Winsvold, Reference Andreassen and Winsvold2012). The word ‘glacier’, then, when affixed to a name, does not always provide a differentiation between a glacier and a glacier complex.
5.2.2 Availability of synchronous data
Ideally, the ranking of the largest glaciers could be directly derived from a globally homogeneous dataset of glacier outlines from the same reference year. Indeed, this is difficult to achieve as adverse snow and cloud conditions can make it difficult to retrieve satellite imagery for the same year for all glacierized regions in the world. Historically, the first, nearly complete, global inventory only became available with RGI 1.0 in 2012 (Pfeffer and others, Reference Pfeffer2014), so it was difficult to answer the question about the world's largest glaciers before then. RGI 6.0 – used for this study – was published in 2017 and provides a snapshot inventory, mostly for the beginning of the 21st century. However, its glacier outlines still span several decades in some regions and more than half a century in the most extreme case. This timing issue can influence rankings due to glacier area changes, which currently range from close to zero to several percent per year depending on the region (Vaughan and others, Reference Vaughan2013; Cogley, Reference Cogley2016; Zemp and others, Reference Zemp2019). In most cases, this will not affect the ranking since relative area change is typically smallest for larger glaciers (e.g. Yang and others, Reference Yang, Hock, Kang, Shangguan and Guo2020). However, the ranking of ice complexes may change if an ice complex is abruptly split into two or more smaller complexes when the connections between individual outlet glaciers are lost due to retreat (Fig. 5).
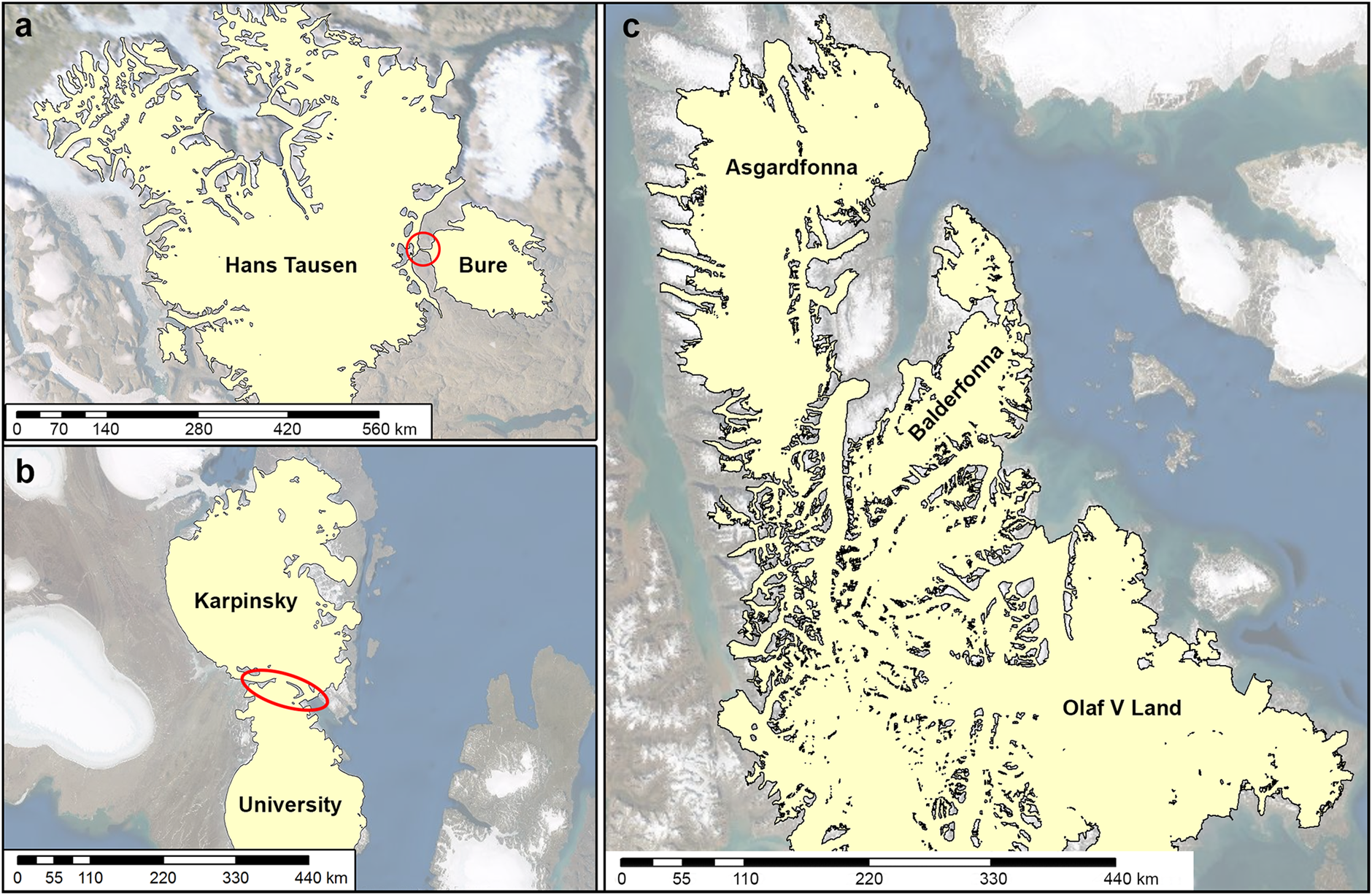
Fig. 5. Examples of glacier complexes with limited connections. (a) Hans Tausen Ice Cap and Bure Ice Cap in Greenland; (b) Karpinskiy Ice Cap and University Ice Cap on October Revolution Island, Severnaya Zemlya; (c) Asgardfonna, Balderfonna and Olaf V Icefields on Svalbard, Norway. Red circles highlight small glacier confluences connecting the ice caps; the background image is the ESRI World Imagery base map (ESRI, 2022).
In addition, asynchronous glacier outlines can have a major effect on glacier ranking when glaciers change rapidly due to dynamically induced retreats or advances, for example, caused by rapid tidewater glacier retreats (McNabb and Hock, Reference McNabb and Hock2014) or surge or tidewater advances (Sevestre and Benn, Reference Sevestre and Benn2015; Brinkerhoff and others, Reference Brinkerhoff, Truffer and Aschwanden2017). In the present study, we tried to minimize the temporal spread by complementing RGI 6.0 with GLIMS, which contains some more recent outlines.
5.2.3 Data quality
Ranking glaciers by area depends also on the uncertainty in the outlines used. Glacier extents can be overestimated due to wrongly mapped seasonal snow, underestimated due to missed glacier ice in shadowed areas, or both over and under estimated when debris cover on glaciers is wrongly interpreted. In general, uncertainties decrease as glacier size increases and are on the order of a few percent for glaciers with a size of a few square kilometers (Paul and others, Reference Paul2013; Raup and others, Reference Raup2014). Thus, for the largest glaciers in a region, this only plays a minor role. More severe is the impact of the quality of the available DEMs on glacier extent. Elevation errors, especially in relatively flat accumulation zones, can have major effects on the location of the automatically computed ice divides, which influence the separation of glacier complexes into glaciers.
Mapping precision is most relevant for glaciers with complex outlines and for very small glaciers. When size differences are smaller than a few percent, the uncertainties become significant, and hence, the related glaciers may share the same rank. Consequently, the Asgardfonna-Balderfonna-Olaf V Glacier Complex (8371 km2) in Svalbard, Vatnajökull (8092 km2) in Iceland and Austfonna Ice Cap (8067 km2) in Svalbard could all be considered to be the second largest glacier complex on the European continent after the Severny Island Northern Ice Cap (20 667 km2) in the Russian Arctic.
5.2.4 Data consistency
Finally, a glacier ranking should ideally be based on a consistent interpretation of glacier extents. In reality, GLIMS and RGI are a compilation of regional or national glacier inventories that originate from different investigators with various implementations of glacier definitions, data sources (platform, sensor and media) and time periods. As a consequence, our glacier ranking might be impacted by interpretation differences across regions.
For glacier complexes, subjective decisions in the mapping of outlines can result in major differences in glacier area and, hence, influence our glacier ranking. For example, the Hans Tausen Ice Cap – the second largest glacier complex in Greenland – is connected with the Bure Ice Cap through a relatively small glacier confluence in the ablation region (Fig. 5a). Separating the two ice caps would reduce the total area of the Hans Tausen glacier complex from 4114 to 3721 km2 but, in this case, not change the ranking in Greenland. A similar situation is seen with the Karpinskiy and University Ice Caps in the Russian Arctic (Fig. 5b). Here, separating the two ice caps would still keep Karpinskiy Ice Cap as the third largest glacier complex. In Svalbard, the largest glacier complex is formed by the Asgardfonna, Balderfonna and Olaf V icefields. All three are connected in the ablation region through common outlet glaciers (Fig. 5c) but regionally often considered as individual ice bodies (Liestøl, Reference Liestøl, Williams and Ferrigno1993), as they are dynamically independent and have separate accumulation zones. Thus, the corresponding glacier complex ranks first in this region. Separating these connections would result in different rankings for the complexes. Without separation, the largest glacier complexes in Svalbard are the Asgardfonna-Balderfonna-Olaf V Glacier Complex (8371 km2), Austfonna Ice Cap (8067 km2) and Holtedalfonna-Isachsenfonna Glacier Complex (5377 km2). Were the glacier complexes separated, the largest would become Austfonna Ice Cap (8067 km2), Vestfonna Ice Cap (2372 km2) and Asgardfonna Icefield (1587 km2). For the purposes of this study and for consistency across all regions, we have chosen not to separate the glacier complexes by these connections in order to show the size of the largest contiguous ice bodies in the world based on the currently available databases.
For individual glaciers, the largest source of inconsistency most likely comes from the division of glacier complexes in the original inventories. Similarly, digital outlines for many ice caps and icefields are only mapped as single glacier complexes but not, or only partly, divided into glaciers. For example, the Vatnajökull Ice Cap in Iceland is stored as ~30 glaciers in RGI 6.0 but as one single ice cap in GLIMS (Fig. 1b). We have chosen to use the individual outlines for glacier rankings as provided in the RGI dataset (the blue lines in Fig. 1b) and are aware that this is somewhat inconsistent. In other regions, in particular the Antarctic and Subantarctic Islands, many glacier complexes are not, or are insufficiently, divided into glaciers. Hence, this may result in glaciers that are inappropriately too large in our ranking. Further, we chose to use connectivity levels 0 and 1 from Rastner and others (Reference Rastner2012) for the Greenland Periphery and exclude glaciers with connectivity level 2. However, Rastner and others (Reference Rastner2012) is a first attempt at a consistent solution to distinguish glaciers that are separate from the Greenland Ice Sheet; but as they note in their paper, this is not always in agreement with other inventories. Thus, if one were to include glaciers with connectivity level 2, the list of the ten largest glaciers in the world might change slightly.
5.3 Outlook on future work
To improve the ranking of glaciers by area, we recommend that ice caps and icefields be consistently split into individual glaciers in the GLIMS glacier database rather than only stored as glacier complexes as is currently the case for many ice bodies. Separation based on drainage divides should be built on the highest resolution DEMs available, and where possible, be guided by high-resolution ice velocity data to reduce ambiguities. Such a homogenized glacier inventory will allow a largely automated ranking of the world's glaciers. We acknowledge that creating drainage divides can be a considerable effort and, thus, recommend storing the divides in a separate data layer with polygon topology, as already recommended by Paul and others (Reference Paul, Kääb, Maisch, Kellenberger and Haeberli2002), so that it may be used again with a dataset from a different point in time and allow for consistent change assessment. These individual glaciers can then be merged into glacier complexes using suitable methods, thus providing a consistent basis for their ranking.
We recommend that global datasets such as future versions of RGI provide both the inventory of individual glaciers as well as a derived inventory of glacier complexes. In addition, rankings should be derived from outlines as close as possible to a common reference year, and thus global inventories should strive for consistency in outline dates at least over a period of ~5–10 years. We acknowledge that this can be difficult to achieve in many regions with adverse cloud conditions and long-lasting seasonal snow where suitable images are simply not available. Storage of multi-temporal outlines of glaciers with common reference years will allow for future work that investigates how rankings change through time as glaciers respond to climate change. Lastly, as glacier inventory data become more internally consistent, this and other such global analysis tasks can be further automated and our analysis can be extended to rankings with respect to glacier length (Machguth and Huss, Reference Machguth and Huss2014) or volume and mass (Huss and Farinotti, Reference Huss and Farinotti2012; Farinotti and others, Reference Farinotti2019).
6. Conclusions
The question of which glaciers are the largest in the world has not been previously answered. Here, we revisited established terminology and used available inventories (i.e. GLIMS and RGI) to provide a systematic and reproducible ranking, differentiated for the seven geographical continents and the 19 first-order glacier regions. A basic requirement for such a ranking is delimiting glacier boundaries based on hydrological basins as well as having a clear differentiation between glaciers and glacier complexes.
We find that the largest glacier complexes cover areas larger than 10 000 km2, exceed the size of the largest glaciers by one order of magnitude, and are located in the polar regions and in the Southern Andes. The largest glacier complexes cover areas the size of smaller countries (such as Bhutan or Austria) but are still orders of magnitudes smaller than the Greenland and Antarctic Ice Sheets and their drainage basins.
Ranking glaciers is highly uncertain and depends on the way the ice is divided, which is subjective and depends on the quality of available data. Ranking requires not only clearly defined glacier terminology but also depends on the availability, quality and consistency of digital glacier outlines at a global scale. Consequently, our rankings are most consistent for glacier complexes and within regions but are subject to larger uncertainties for glaciers and across regions.
Supplementary material
The supplementary material for this article can be found at https://doi.org/10.1017/jog.2022.61
Data
Access to the digital glacier outlines is provided by NSIDC for the largest glaciers from this study (https://doi.org/10.7265/0k6h-yn09; Windnagel and Zemp, Reference Windnagel and Zemp2022), RGI 6.0 (https://doi.org/10.7265/N5-RGI-60; RGI Consortium, 2017) and the GLIMS V20190304 database (https://doi.org/10.7265/N5V98602; GLIMS Consortium, 2005). The outlines of the 19 glacier regions are available from the GTN-G (https://doi.org/10.5904/gtng-glacreg-2017-07).
Code availability
Acknowledgements
We thank Florentin Brendler, University of Innsbruck, for initial work on this topic as WGMS intern and Dorian Delnon, University of Zurich, for support with the design of Figures 2, 3, and 4. We also thank Florence Fetterer and Todd Johnston of NSIDC and the Journal of Glaciology reviewers and editors for reviewing the manuscript and providing valuable feedback. The present study was carried out as a joint project by the US National Snow and Ice Data Center (NSIDC) and the World Glacier Monitoring Service (WGMS) and is a contribution to the Working Group on the ‘RGI and its role in future glacier monitoring and GLIMS’ of the International Association of Cryospheric Sciences (IACS). This work was enabled by support from the US National Oceanic and Atmospheric Administration (NOAA) cooperative agreement with the Cooperative Institute for Research in Environmental Sciences (CIRES) at the University of Colorado Boulder under grant number NA17OAR4320101, the Federal Office of Meteorology and Climatology MeteoSwiss within the framework of the Global Climate Observing System (GCOS) Switzerland, the Copernicus Climate Change Service (C3S) implemented by the European Centre for Medium-range Weather Forecasts (ECMWF) on behalf of the European Commission, the European Space Agency (ESA) projects Glaciers_cci (4000127593/19/I-NB), NASA (grants 80NSSC20K1296 and 80NSSC17K0566), and the Research Council of Norway (RCN, grant #324131).