Introduction
Major depressive disorder (MDD) is one of the leading causes of disability worldwide (Vos et al., Reference Vos, Allen, Arora, Barber, Bhutta, Brown and Murray2016). Adolescence is a critical period for the development of depression with a cumulative incidence for first-onset depression of about 36% among female and 14% among male teenagers (12–17 years of age; Breslau et al., Reference Breslau, Gilman, Stein, Ruder, Gmelin and Miller2017). The worldwide prevalence of any depressive disorder in this age group is estimated at 2.6% (Polanczyk, Salum, Sugaya, Caye, & Rohde, Reference Polanczyk, Salum, Sugaya, Caye and Rohde2015). Around 40% of adolescents do not benefit from the available first- and second-line treatments (i.e. psychotherapy and/or pharmacotherapy; Birmaher & Brent, Reference Birmaher and Brent2007). Vagus nerve stimulation (VNS) is a promising third-line therapeutic option for treatment-resistant depression in adults (Aaronson et al., Reference Aaronson, Sears, Ruvuna, Bunker, Conway, Dougherty and Zajecka2017). Recent technological advances allow for the transcutaneous stimulation of the vagus nerve (tVNS), minimizing the risk associated with surgery for implanting the stimulator. In adults, tVNS has been shown to reduce depressive symptoms following 4 weeks of stimulation (Fang et al., Reference Fang, Rong, Hong, Fan, Liu, Wang and Kong2016). The potential of tVNS in the treatment of underage patients with depression has not yet been explored.
In a pre-clinical experimental trial, we aimed to investigate the potential effects of acute tVNS on neuropsychological proxies of MDD, namely emotion recognition. Deficits in recognizing facial expressions of emotional valence across all basic emotions except sadness have been repeatedly reported in depressed patients (Dalili, Penton-Voak, Harmer, & Munafò, Reference Dalili, Penton-Voak, Harmer and Munafò2015). Regarding sad facial expressions, patients with MDD show a negativity bias (e.g. tendency to rate natural stimuli as negative). In adults, selective serotonin reuptake inhibitors (SSRI) have been shown to improve emotion recognition (Harmer et al., Reference Harmer, Bhagwagar, Perrett, Völlm, Cowen and Goodwin2003) and changes in processing facial expressions following SSRI administration have been shown to predict later treatment outcome (Godlewska, Browning, Norbury, Cowen, & Harmer, Reference Godlewska, Browning, Norbury, Cowen and Harmer2016). Behavioral data in healthy adults suggest similar effects of tVNS action as tVNS enhances the recognition of emotions in faces (Sellaro, de Gelder, Finisguerra, & Colzato, Reference Sellaro, de Gelder, Finisguerra and Colzato2018) and promotes the ability to decode salient social cues (Colzato, Sellaro, & Beste, Reference Colzato, Sellaro and Beste2017). In a very first study with underage depressed patients, we aimed to study the potential antidepressant effects of tVNS by assessing its acute effects on emotion recognition.
Materials and methods
General procedures
The ethics committee of the Medical Faculty, Heidelberg University approved the study (Study ID: S-297/2016 and S-365/2017). The trial was registered at the German Clinical Trials Register (Study ID: DRKS00011112) and the World Health Organization (Universal Trial Number: U1111-1188-0829). Adolescent patients with MDD during a current depressive episode were recruited consecutively at the Clinic for Child and Adolescents Psychiatry, Heidelberg University between December 2016 and October 2017. Non-depressed controls were recruited between October 2017 and January 2018 via public advertisement. Due to ethical and legal restrictions, non-depressed controls were adolescents fulfilling diagnostic criteria for tension-type headache (TTH). The tVNS device used (see below) has a CE marking for the treatment of depression and pain in accordance with EU regulations regarding conformity with health, safety, and environmental protection standards. Thus, we were not allowed to conduct an experimental trial with completely healthy controls, not fulfilling the area of intended indication according to CE criteria. All participants and their legal guardians provided written informed consent before inclusion in the study. Participants received an allowance of €50 for participation. Details on the inclusion and exclusion criteria as well as the participant flow are reported in the online Supplementary Material. The general study design is illustrated in Fig. 1.

Fig. 1. Illustration of the study design; the study comprised two appointments. Diagnostic assessments were conducted at T1. The actual experiment, including the neuropsychological tasks, was conducted at T2. Illustrated are the tVNS/sham stimulation periods (randomized order across participants); recording periods for electrocardiography (ECG), electrodermal activity (EDA) as well as function near infrared spectroscopy (fNIRS); and time points of saliva sampling and self-reports on current affective states (PANAS).
Clinical assessments and self-reports
Following an initial screening, participants were informed about the study details. In case written informed consent was obtained, participants were assigned a study ID and invited to a first appointment including all clinical assessments (T1). At T1, participants provided basic sociodemographic information before completing several clinical interviews. All assessments were parallelized across groups. Clinical interviews included the German version of the Mini-International Neuropsychiatric Interview for Children and Adolescents (M.I.N.I – KID 6.0; Sheehan et al., Reference Sheehan, Sheehan, Shytle, Janavs, Bannon, Rogers and Wilkinson2010). Patients had to endorse diagnostic criteria for MDD with a current depressive episode. Non-depressed controls were only included if they did not endorse any primary psychiatric disorder requiring treatment or any current psychiatric or psychological treatment. Participants completed the Children's Depression Rating Scale – Revised (CDRS-R; Poznanski, Freman, & Mokros, Reference Poznanski, Freman and Mokros1985). Only patients scoring above the severity cut-off score of 34 were included in the trial. Controls scoring above the cut-off were excluded. Clinicians rated the level of emotional and behavioral functioning in the past 3 months using the German version of the Children's Global Assessment Scale (C-GAS; Shaffer et al., Reference Shaffer, Gould, Brasic, Ambrosini, Fisher, Bird and Aluwahlia1983). Participants were asked to complete several self-reports, including the Difficulties in Emotion Regulation Scale (DERS; Gratz & Roemer, Reference Gratz and Roemer2004), and the Beck Depression Inventory II (BDI-II; Beck, Steer, & Brown, Reference Beck, Steer and Brown1996; Osman, Kopper, Barrios, Gutierrez, & Bagge, Reference Osman, Kopper, Barrios, Gutierrez and Bagge2004). At T2, patients further completed the State Trait Anxiety Inventory (STAI; Laux, Glanzmann, Schaffner, & Spielberger, Reference Laux, Glanzmann, Schaffner and Spielberger1981). The assessment further included other clinical interviews and self-reports (details reported in the online Supplementary Material), not reported in the present manuscript. All interviews and self-reports were computerized using LimeSurvey.
Neurobiological measures
Following interviews and self-reports at T1, functional near-infrared spectroscopy (fNIRSFootnote †Footnote 1) of the prefrontal cortex, electrocardiography (ECG), and electrodermal activity (EDA) were recorded during a 5 min baseline while participants completed a Color Detection Task (CDT; Jennings, Kamarck, Stewart, Eddy, & Johnson, Reference Jennings, Kamarck, Stewart, Eddy and Johnson1992; see online Supplementary Material). After the baseline, a saliva sample was taken. Time stamps for all events were recorded on computerized paper (TeleForm–Electric Paper). Participants then received 5 min of tVNS and sham stimulation in randomized order while fNIRS, ECG, and EDA were continuously recorded. At the end of T1, weight and height were measured. Data from physiological recordings at T1 are not reported. Following T1, eligible patients were invited to a second appointment. All appointments for T1 and T2 were scheduled in the afternoon (past 1 pm). After completion of self-reports, equipment for the recording of fNIRS, ECG, and EDA was attached. Patients again first completed a 5 min baseline (CDT) after which the tVNS stimulator was attached. Patients then completed a 15 min pre-stimulation phase (tVNS or sham) while watching a video (see online Supplementary Material) followed by the first task period, and a 10 min postline (CDT). Baseline (5 min), pre-stimulation (tVNS or sham; 15 min video), task-period, and post-line (10 min, CDT) were repeated before participants were debriefed. fNIRS, ECG, and EDA were continuously recording throughout all procedures at T2. Participants provided saliva samples after each baseline, after each pre-stimulation period, after each task-period, and after each postline. While providing saliva samples, participants completed self-reports on positive and negative affect using a computerized short version of the Positive and Negative Affect Schedule (PANAS; Watson, Clark, & Tellegen, Reference Watson, Clark and Tellegen1988) and rated their perceived stress and current mood on 100 mm visual analogue scales (VAS). Focusing on measures quantifying physiological effects of tVNS on autonomic nervous system activity, saliva samples were used for the assay of α-amylase. ECG data were analyzed to quantify heart rate variability (HRV) and mean heart rate (HR) for each segment. EDA data were analyzed to derive skin conductance response (SCR). Here we only report on α-amylase and SCR as proxies of sympathetic activity, HRV as a proxy of parasympathetic activity and HR (mixed influence). fNIRS was recorded as an additional measure to quantify oxygenation in the prefrontal cortex (not reported). Details on the recording and analyses of all neurobiological measures are provided in the online Supplementary Material.
Transcutaneous vagus nerve stimulation
tVNS was applied using the VITOS® t-VNS device (Cerbomed, Erlangen, Germany). The VITOS® is a battery powered handheld stimulator with an ear electrode stimulating at the concha of the outer ear. It has a CE mark for the treatment of pain and depression (CE0408). Stimulation is achieved via a series of electrical pulses with a pulse width of 250 µs at a frequency of 1 Hz with a cycle of 30 s on and 30 s off to avoid habituation. The stimulation intensity for all participants and both conditions (tVNS and sham) was set to 0.5 mA. Before placement of the ear electrode, the left concha and ear lobe were cleaned using alcohol swipes (70% isopropyl alcohol). During active tVNS, the ear electrode was placed in contact with the skin of the left concha, as illustrated in Fig. 2a. For sham stimulation, the ear electrode was placed in contact with the left ear lobe, not innervated by the vagus nerve (Burger et al., Reference Burger, Verkuil, Van Diest, Van der Does, Thayer and Brosschot2016; Colzato et al., Reference Colzato, Sellaro and Beste2017; Frangos, Ellrich, & Komisaruk, Reference Frangos, Ellrich and Komisaruk2015) as illustrated in Fig. 2b. The device automatically measures impedance and insufficient contact of the electrode with the skin evokes an alarm. tVNS or sham stimulation were applied in a within-subject cross-randomized order. tVNS or sham stimulation started 15 min (pre-stimulation) before participants were asked to complete the neuropsychological tasks.
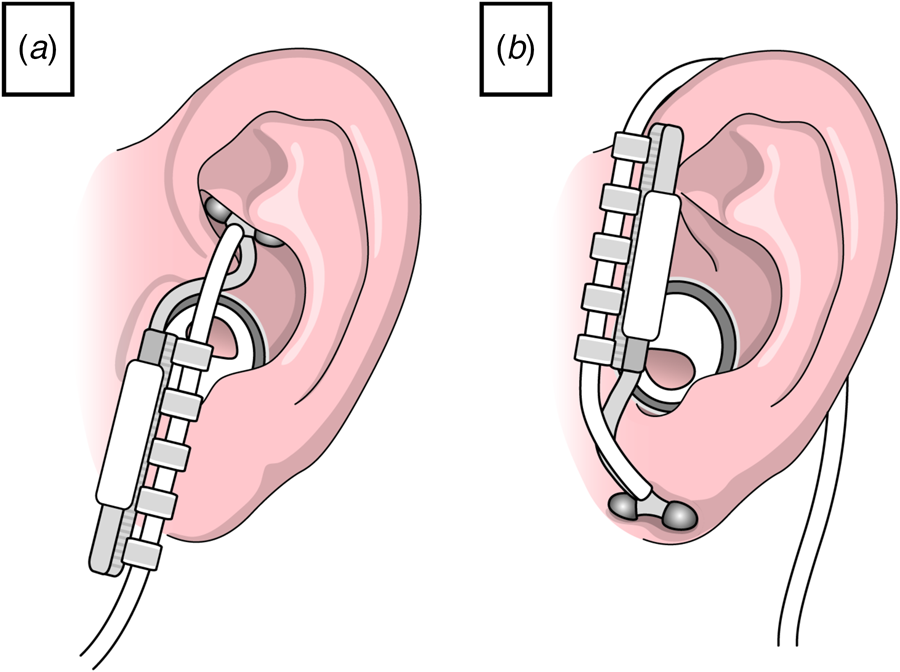
Fig. 2. Schematic illustration of the placement of the ear electrode; (a) active transcutaneous vagus nerve stimulation; the ear electrode is placed in contact with the skin of the left concha; (b) sham stimulation; the ear electrode is placed in contact with the left ear lobe, not innervated by the vagus nerve.
Neuropsychological tasks
A total of three computerized neuropsychological tasks were used to quantify different facets of emotion recognition. All tasks were programmed using PsychoPy (version: 1.84; Peirce, Reference Peirce2007). Stimuli for the different tasks were taken from the FACES database, a database of facial expressions in younger, middle-aged, and older women and men (Ebner, Riediger, & Lindenberger, Reference Ebner, Riediger and Lindenberger2010). Only faces of younger and middle-aged women and men were used (the tasks including lists of stimuli are available online: https://osf.io/pjnhx/). All tasks were programmed in two versions (A/B) for repeated presentation, using distinct sets of stimuli. The order of tasks was kept constant, while the order of versions was randomized. Stimuli were randomly assigned to the different tasks to avoid the repeated presentation of the same faces, except for the emotional Go/NoGo-task. Age (younger and middle-aged) and sex (women and men) of faces were equally balanced across tasks, versions (A/B), and emotional expressions. The reporting of all methodological details of the tasks is provided in the online Supplementary Material. First, patients were asked to complete a task assessing the capacity to recognize emotions (happy, sad, anger, fear) in gradually changing facial expressions (starting from neutral toward the target emotion). Patients were instructed to press the mouse key once they recognize the expressed emotion. The response time (time until recognition of emotional expression and selection of one category) in ms, the level of emotion expression at response in percent, and the selected category (correct responses) were recorded. Second, patients were asked to complete a basic emotion recognition task. Participants were asked to select the category of the expressed static emotion (happy, neutral, sad), and in case participants selected one of the discrete emotional categories (happy or sad), they were asked to rate the intensity of the expressed emotion (‘How intense is the expressed emotion?’) on a 100 mm VAS. The response time (time until selection of one category) in ms, the selected category (correct responses), and the rating of emotional intensity (0–100; in case happy or sad were selected as category) were recorded. Third, patients were asked to complete an emotional Go/NoGo-task designed in accordance with a previous study (Trinkl et al., Reference Trinkl, Greimel, Bartling, Grünewald, Schulte-Körne and Grossheinrich2015). Following an instruction screen, patients were asked to press the left mouse key whenever a target stimulus was displayed (go-cue) and inhibit the response whenever a non-target stimulus was displayed (no-go-cue). Each version of the task (A/B) consisted of two blocks. Across blocks go- and no-go-cues were interchanged. The number of correct and false hits, as well as the reaction time in ms, was recorded.
Statistical analyses
Differences between groups on sociodemographic and clinical variables were analyzed using χ2 (categorical variables) or t tests (continuous variables), respectively. Neuropsychological task data were analyzed using multilevel mixed-effects generalized linear models for binominal data (dichotomous outcomes) or multilevel mixed-effects linear regression (continuous outcomes). Group (MDD v. controls) and condition (tVNS v. sham) as well as their interaction were addressed as fixed effects. The participants' ID was entered as a random effect. In the case of a significant group by condition interaction, we further tested for the effect of target emotion (e.g. sad v. happy) in each level of the interaction. Self-reports (stress, mood, positive, and negative effect) as well as physiological data (HR, HRV, SCR, α-amylase) were analyzed using multilevel mixed-effects linear regression with time (segment or time of measurement), group, and condition as fixed effects and the participants' ID as random effect. Based on reviewer recommendations, we performed additional analyses. (1) In line with previous tVNS research (Colzato et al., Reference Colzato, Sellaro and Beste2017; Sellaro et al., Reference Sellaro, de Gelder, Finisguerra and Colzato2018), we conducted additional analyses addressing a potential interaction of tVNS stimulation with item difficulty (i.e. recognizability of faces). Data on item difficulty were derived from a validation study as provided on the FACES website (http://faces.mpdl.mpg.de/imeji/). In line with previous studies (Colzato et al., Reference Colzato, Sellaro and Beste2017; Sellaro et al., Reference Sellaro, de Gelder, Finisguerra and Colzato2018), item difficulty was dichotomized to compare easy and difficult items. (2) In exploratory analyses, we addressed the linear association between effects seen under tVNS and measures of clinical severity on a continuum using Pearson's correlations (two sided). All analyses were performed using Stata (Version 15.1; StataCorp LP, College Station, TX, USA), at an α level of 0.05. All contrasts were Sidak corrected.
Results
Sample characteristics
Groups did not differ on sex, age, height, weight, or school-type. Groups differed on all clinical variables, with MDD patients reporting greater depression severity, greater difficulties in emotion regulation, state and trait anxiety, as well as a lower level of functioning. Detailed reporting on group differences in sociodemographic and clinical variables is provided in Table 1.
Table 1. Sociodemographic and clinical characteristics by group

All values are means and standard deviations (s.d.) in brackets unless otherwise indicated; MDD, major depressive disorder; school, after 4 years of elementary school the German school system branches into three types of secondary schools. The so-called Hauptschule (Secondary General School which takes 5 years after Primary School) prepares pupils for vocational training, whereas the Realschule (Intermediate Secondary School) concludes with a general certificate of secondary education after 6 years. Eight years of Gymnasium provide pupils with a general university entrance qualification; medication: multiple counts possible, data on doses and duration of intake available upon request; CDRS, Children's Depression Rating Scale – Revised; BDI, Beck Depression Inventory II; DERS, Difficulties in Emotion Regulation Scale; STAI, State Trait Anxiety Inventory; CGAS, Children's Global Assessment Scale; no missing data; *comorbidity in controls: harmful use of alcohol (F10.1; n = 4); remitted depressive disorder (F33.4; n = 3); social phobia (F40.1; n = 2); agoraphobia with panic disorder (F40.01; n = 1).
Self-reported mood and stress
Positive affect [χ2(7) = 97.36, p < 0.0001], negative affect [χ2(7) = 21.22, p = 0.004], stress [χ2(7) = 44.52, p < 0.0001], and current mood [χ2(7) = 27.66, p < 0.001] varied over time. Further, positive affect [χ2(1) = 34.94, p < 0.0001], negative affect [χ2(1) = 21.14, p < 0.0001], stress [χ2(1) = 37.74, p < 0.0001], and current mood [χ2(1) = 74.13, p < 0.0001] differed as a function of group, indicating less stress and negative affect as well as increased positive affect and current mood in controls compared to patients with MDD. There were no significant main effects of tVNS. There was a significant group by tVNS interaction on ratings of current mood [χ2(1) = 9.27, p = 0.002], such that mood slightly decreased (−3.45 points on the VAS) under tVNS in patients with MDD, mainly in the respective baseline and pre-stimulation phases. There were no effects in controls. Findings are illustrated in online Supplementary Fig. S1.
Emotion recognition
Groups significantly differed in the time needed to classify and correctly classify gradually expressed emotions (Task 1). Controls were faster in classifying gradually changing facial expressions of emotions. Similarly, groups significantly differed in the time needed to classify and correctly classify static expressed emotions (Task 2). Controls were faster in classifying static facial expressions of emotions. There were no effects of tVNS on the recognition of static or gradually expressed emotions (see Table 2).
Table 2. Descriptive statistics on neuropsychological task outcomes by group and condition

MDD, major depressive disorder; tVNS, transcutaneous vagus nerve stimulation; sham, sham stimulation; hits, correct responses/correctly classified emotions; response time, time until response/recognition; intensity, ratings of intensity for discrete emotions on a visual analogue scale (VAS) 0–100; correct trials, only considering data when the emotion was correctly classified; omission errors, no reaction toward a target stimulus (go-trial); commission error, reaction toward a non-target stimulus (nogo-trial); percentage measures on correct hits only provided for descriptive purposes, analyses were based on dichotomous data nested by subject and trial using multilevel mixed-effects generalized linear models.
Groups significantly differed on correct responses and omission errors in the Go/NoGo-task. Controls were more likely to respond correctly and made less omission errors. tVNS had a significant effect on correct responses and omission errors, such that sham stimulation was associated with less correct responses and more omission errors. Further, there was a significant group by tVNS interaction on correct responses and omission errors, such that correct responses increased under tVNS in controls, while decreasing under tVNS in patients with MDD. Omission errors increased under tVNS in patients with MDD and decreased under tVNS in controls (Table 2), as illustrated in Fig. 3.

Fig. 3. Main effects of tVNS on emotion recognition (hits and omission errors) in the emotional Go/NoGo-task by group; hits, correct responses toward target-stimuli in percent (total of 128 stimuli per condition); omission errors, no reaction toward a target stimulus (go-trial) in percent; tVNS, transcutaneous vagus nerve stimulation at the concha of the left outer ear; sham, sham-stimulation of the left ear lobe; MDD, adolescents with major depression; control, non-depressed adolescents; illustrated are means and 95% confidence intervals.
Analysis in each level of the significant group by tVNS interaction showed that the effects differed as a function of target emotion. In patients with MDD, correct hits on sad emotions decreased under tVNS (go-negative: z = −4.36, p < 0.0001) and no effects occurred for happy faces (go-positive: z = 0.78, p = 0.437). In controls, correct hits on happy (go-positive: z = 4.63, p < 0.0001) and sad emotions (go-negative: z = 2.31, p = 0.021) increased under tVNS. Omission errors on sad target stimuli (go-negative: z = 5.79, p < 0.0001) increased under tVNS in patients with MDD, and showed no differences for happy target stimuli (go-positive: z = −0.41, p = 0.685). In controls, omission errors decreased on sad (go-negative: z = −2.50, p = 0.012) and happy (go-positive: z = −6.56, p < 0.0001) target emotions under tVNS. Findings are illustrated in Fig. 4.

Fig. 4. Effects of tVNS on emotion recognition (hits and omission errors) in the emotional Go/NoGo-task by target emotion and group; hits, correct responses toward target-stimuli in percent (total of 64 stimuli per condition and emotion); omission errors, no reaction toward a target stimulus (go-trial) in percent (%); tVNS, transcutaneous vagus nerve stimulation at the concha of the left outer ear; sham: sham-stimulation of the left ear lobe; MDD, adolescents with major depression; control, non-depressed adolescents; go-negative, target stimuli presenting faces with sad expressions; go-positive, target stimuli presenting faces with happy expressions; illustrated are means and 95% confidence intervals.
Autonomic nervous system function
α-amylase [χ(7) = 26.58, p < 0.001] changed over time but there were no significant main effects of group [χ(1) = 1.66, p < 0.198] or condition [χ(1) = 1.62, p < 0.202]. Both HR [χ(17) = 376.03, p < 0.0001] and HRV [χ(17) = 249.97, p < 0.0001] changed over time. HR [χ(1) = 6.92, p = 0.009] and HRV [χ(1) = 15.21, p < 0.0001] showed main effects of group, indicating lower HR and greater HRV in controls compared to patients with MDD. HR [χ(1) = 0.72, p = 0.395] and HRV [χ(1) = 2.03, p = 0.154] showed no main effects of tVNS. However, there were significant tVNS by group interactions on HR [χ(1) = 13.33, p < 0.001] and HRV [χ(1) = 14.33, p < 0.001]. HR decreased and HRV increased in controls under tVNS. There were no effects in patients with MDD. SCR changed over time [χ(17) = 119.73, p < 0.0001], but showed no significant main effect of group [χ(1) = 0.15, p = 0.694] or tVNS [χ(1) = 0.35, p = 0.553]. Findings are illustrated in online Supplementary Fig. S2.
Additional analyses: item difficulty and clinical severityFootnote 2
Item difficulty (easy v. difficult) had a significant main effect on correct responses [χ(1) = 4.86, p = 0.027] and time to correctly identify emotions [χ(1) = 4.99, p = 0.025] in the recognition of gradually expressed emotions. Similarly, item difficulty had a significant main effect on correct responses when identifying static expressed emotions [χ(1) = 5.00, p = 0.025] and on the perceived intensity of expressed emotions [all items: χ(1) = 32.71, p < 0.0001; correctly identified items: χ(1) = 50.57, p < 0.0001]. However, item difficulty showed no significant interaction with stimulation condition (tVNS v. sham) or group (MDD v. controls). Item difficulty had no significant effect on any outcome of interest in the emotional Go/NoGo task.
Finally, we addressed the linear association between effects seen under tVNS in the emotional Go/NoGo task and measures of clinical severity. We found a significant association between the change in correct responses under tVNS and depression severity [CDRS interview: r (63) = −0.272, p = 0.031; BDI self-reports: r (63) = −0.253, p = 0.046], as well as self-reports on difficulties in emotion regulation [DERS: r (63) = −0.261, p = 0.039]. Regarding omission errors, we found similar associations between changes under tVNS and depression severity [CDRS interview: r (63) = 0.319, p = 0.011; BDI self-reports: r (63) = 0.289, p = 0.021], difficulties in emotion regulation [DERS: r (63) = 0.294, p = 0.020], and the global level of functioning [CGAS: r (63) = −0.266, p = 0.035]. The later findings are illustrated in Fig. 5. Findings suggest that patients with greater depression severity, greater difficulties in emotion regulation and lower functioning showed the greatest effects of tVNS.

Fig. 5. Linear associations between effects of tVNS and measures of a clinical outcome; illustrated are changes in omission errors under tVNS (delta score: sham – tVNS) in association with the respective clinical outcome in the full sample. omission errors, no reaction toward a target stimulus (go-trial) in percent (%); CDRS, Children's Depression Rating Scale – Revised; BDI, Beck Depression Inventory II; DERS, Difficulties in Emotion Regulation; CGAS, Children's Global Assessment Scale; blue dots: patients with MDD, gray dots: non-depressed controls.
Discussion
The present study is the first to investigate the effects of acute tVNS on emotion recognition in adolescents with MDD. tVNS had no robust effects on self-reports of mood or affect and no effects on the recognition of static or gradually increasing facial expressions of emotions. The recognition of briefly presented stimuli in the Go/NoGo-task increased under tVNS in controls but decreased in patients with MDD. Additional analyses indicated that beyond group effects, there was a linear association between changes in emotion recognition under tVNS and different continuous measures of depression severity and associated symptoms (difficulties in emotion regulation, general functioning). Sensitivity analyses indicated that tVNS specifically led to a decrease in the recognition of sad emotions in patients with MDD. In controls, tVNS increased the recognition of happy and sad emotions. Whereas tVNS generally improves emotion recognition in controls, it seems to specifically decrease the recognition of emotions of negative valence in adolescent MDD.
Findings in non-depressed controls are in line with previous research in adults, suggesting that tVNS enhances the general ability to recognize emotions (Sellaro et al., Reference Sellaro, de Gelder, Finisguerra and Colzato2018). However, unlike previous studies presenting static stimuli with no response-time limitation, we found tVNS to only affect early visual processing and subsequent behavioral responses to briefly presented stimuli of emotional valence. Even when controlling for item difficulty in line with previous tVNS studies (Colzato et al., Reference Colzato, Sellaro and Beste2017; Sellaro et al., Reference Sellaro, de Gelder, Finisguerra and Colzato2018), we were not able to replicate the reported effects of tVNS on emotion recognition in healthy adults. Findings in adolescent patients with MDD suggest an increase of valence-specific errors, such that tVNS resulted in an impairment to recognize stimuli of negative valence. The strength of this effect varied as a linear function of depression severity (interview and self-reports), self-reported difficulties in emotion regulation, and clinician-rated level of functioning. Findings suggest that patients with greater symptom severity may show enhanced benefit from tVNS stimulation. These results highlight a potential therapeutic mechanism of tVNS by reducing the attentional bias toward stimuli of negative valence in MDD (Gotlib & Joormann, Reference Gotlib and Joormann2010). A previous neuroimaging study in healthy adults using carotid stimulation to evoke enhanced parasympathetic activity showed similar effects, such that amygdala activity was exclusively affected by carotid stimulation during the appraisal of fearful (not neutral) faces (Makovac et al., Reference Makovac, Garfinkel, Bassi, Basile, Macaluso, Cercignani and Critchley2015). Somewhat puzzling is the fact that this effect is only seen in patients with MDD. Non-depressed controls showed a general improvement on the Go/NoGo task. This might be due to the specific nature of our control group, as adolescents with TTH might have a general deficit in emotion recognition that improves under tVNS. On the other hand, tVNS may have resulted in a general enhancement of emotion recognition as suggested by others (Colzato et al., Reference Colzato, Sellaro and Beste2017; Sellaro et al., Reference Sellaro, de Gelder, Finisguerra and Colzato2018) when no prior deficit is present.
It has been shown that antidepressant effects of SSRI administration are attributable to an augmentation of serotonin that enhances pleasant and suppresses unpleasant cortical processing of emotionally valent stimuli (Kemp, Gray, Silberstein, Armstrong, & Nathan, Reference Kemp, Gray, Silberstein, Armstrong and Nathan2004). Although the neurobiological mechanisms of tVNS action are largely unknown, animal studies have shown that VNS increases the firing rate of norepinephrine neurons in the locus coeruleus and serotonin neurons in the dorsal raphe nucleus (Manta, El Mansari, & Blier, Reference Manta, El Mansari and Blier2012). Thus, it is plausible that tVNS exerts its antidepressant action through similar mechanisms of serotonergic action, which remains subject to further investigation. However, here we presented preliminary findings on acute tVNS. Future studies need to address how these could accumulate to meaningful clinical effects following long-term treatment. Neuroimaging studies in adults with MDD receiving 4 weeks of tVNS treatment were able to show that resting state functional connectivity between the right amygdala and left dorsolateral prefrontal cortex was increased in the tVNS compared to the sham condition and that this increase was associated with a reduction in depressive symptoms (Liu et al., Reference Liu, Fang, Wang, Rong, Hong, Fan and Kong2016). Potentially, the short-term effects that we observed translate to changes in neuroplasticity and functional reorganization following longer time periods of tVNS. Respective studies in adolescents are warranted, addressing the clinical utility of tVNS in child and adolescent psychiatric care.
Multi-modal assessments of autonomic nervous system function showed that acute tVNS was associated with an increase in HRV and a decrease of HR only in controls. The absence of effects in patients with MDD suggests that ANS regulation is not susceptible to acute-tVNS, but may require prolonged therapeutic intervention to show meaningful effects (Koenig et al., Reference Koenig, Westlund Schreiner, Klimes-Dougan, Ubani, Mueller, Lim and Cullen2018). In line with the findings of prior meta-analyses (Kemp et al., Reference Kemp, Quintana, Gray, Felmingham, Brown and Gatt2010; Koenig, Kemp, Beauchaine, Thayer, & Kaess, Reference Koenig, Kemp, Beauchaine, Thayer and Kaess2016), patients with MDD from the present sample showed greater HR and lower HRV compared to controls. Findings in non-depressed controls contradict previous studies on the physiological effects of acute tVNS that showed no effects of left-sided but right-sided stimulation on HR/HRV in healthy adults (De Couck et al., Reference De Couck, Cserjesi, Caers, Zijlstra, Widjaja, Wolf and Gidron2017). Differences between studies are potentially attributable to different stimulation parameters (i.e. Hz, duration; De Couck et al., Reference De Couck, Cserjesi, Caers, Zijlstra, Widjaja, Wolf and Gidron2017) that require systematic investigation in future trials. The present findings in non-depressed controls suggest that stimulation of the left vagal nerve tract has small efferent effects on heart function. Effects are characterized by an increase in parasympathetic vagal activity, whereas we found no evidence for changes in sympathetic activity.
The study has some limitations that need to be considered. First, due to legal restrictions, we were not able to recruit a control group of adolescents free of any medical condition. Although the control group consisted of adolescents without depressive symptoms, we cannot rule out the possibility that TTH itself is associated with impaired emotion recognition. TTH has been shown to be associated with emotional distress (Perozzo et al., Reference Perozzo, Savi, Castelli, Valfrè, Lo Giudice, Gentile and Pinessi2005) and is frequently comorbid with depressive symptoms and anxiety (Song et al., Reference Song, Cho, Kim, Yang, Yun and Chu2016). However, we excluded controls with clinically relevant symptoms of depression, and significant group differences on all clinical variables illustrate that we were able to recruit a non-depressed control group to contrast the clinical phenotype of interest. Comparisons against a completely healthy control group – free of any medical condition – might have shown even stronger effects. Thus, the nature of our control group may even enhance confidence regarding the reported effects. Potentially, the nature of the control group and the age range studied are two reasons why we were not able to replicate previous findings of tVNS on emotion recognition reported in healthy adults (Colzato et al., Reference Colzato, Sellaro and Beste2017; Sellaro et al., Reference Sellaro, de Gelder, Finisguerra and Colzato2018). Finally, within this limited sample of adolescents, we were not able to address potential mediating effects of sex, medication status, or other confounds.
Conclusion
To conclude, the present results lend initial support for a potential antidepressant effect of acute tVNS in adolescents with MDD. Future clinical studies are needed, investigating the clinical effects of long-term stimulation on clinical outcomes of depression severity. Further, neuroimaging studies are warranted, addressing potential alterations in cortical processing of emotional stimuli under acute tVNS.
Supplementary material
The supplementary material for this article can be found at https://doi.org/10.1017/S0033291719003490.
Acknowledgements
We would like to thank all the participants and their parents, as well as our clinical staff for their tremendous help in recruiting patients.
Financial support
The project was funded through a Post-Doctoral Scholarship (JK) provided by the Daimler and Benz Foundation (Ladenburg, Germany) and the Thrasher Research Fund Early Career Award provided (JK) by the Thrasher Research Fund (Salt Lake City, UT, USA).
Conflict of interest
The authors have no conflicts of interest to declare.
Ethical standards
The authors assert that all procedures contributing to this work comply with the ethical standards of the relevant national and institutional committees on human experimentation and with the Helsinki Declaration of 1975, as revised in 2008.