1. Introduction
Microtubules are dynamic hollow polymers consisting of 13 straight protofilaments formed by the head-to-tail polymerisation of α,β-tubulin heterodimers. Microtubules become organised to form various arrays to perform suitable cellular functions in need for particular cell types and during cell cycle, including chromosome segregation, cell polarity and cell morphogenesis (Kirschner & Mitchison, Reference Kirschner and Mitchison1986). In plant interphase cells, plasma membrane-associated cortical microtubules guide polar deposition of cellulose microfibrils in newly formed cell walls (Paredez et al., Reference Paredez, Somerville and Ehrhardt2006), and contribute to straight elongation of axial organs, formation of complex cell shapes and development of secondary cell wall patterns (Ehrhardt & Shaw, Reference Ehrhardt and Shaw2006; Lin & Yang, Reference Lin and Yang2020; Oda & Fukuda, Reference Oda and Fukuda2012). Microtubule array may quickly adopt a new pattern or become transiently disassembled in response to external and internal cues, such as light, osmotic stress and phytohormones (Fujita et al., Reference Fujita, Pytela, Hotta, Kato, Hamada, Akamatsu, Ishida, Kutsuna, Hasezawa, Nomura, Nakagami and Hashimoto2013; Lindeboom et al., Reference Lindeboom, Nakamura, Hibbel, Shundyak, Gutierrez, Ketelaar, Emons, Mulder, Kirik and Ehrhardt2013; Shibaoka, Reference Shibaoka1994). Generation, disassembly and maintenance of diverse microtubule arrays are generally governed by microtubule nucleation, microtubule regulators and microtubule-based motor proteins (Hamada, Reference Hamada, Nagasaki-Takeuchi, Kato, Fujiwata, Sonobe, Fukao and Hashimoto2014; Nakamura, Reference Nakamura2014; Nebenführ & Dixit, Reference Nebenführ and Dixit2018). Microtubule severing also greatly contributes to (re)organisation of plant microtubules. In this review, we update previous reviews on plant katanin (Luptovčiak et al., Reference Luptovčiak, Komis, Takáč, Ovecka and Samaj2017; Nakamura, Reference Nakamura2014) and discuss how katanin is targeted to distinct subcellular locations.
2. Katanin severs microtubules
Among related eukaryotic enzymes that generate internal breaks in microtubules, katanin is the sole severing enzyme established in plants (McNally & Roll-Mecak, Reference McNally and Roll-Mecak2018). Katanin consists of a p60 catalytic subunit and a p80 regulatory subunit (Hartman et al., Reference Hartman, Mahr, McNally, Okawa, Iwamatsu, Thomas, Cheesman, Heuser, Vale and McNally1998). The p60 subunit belongs to the ATPase associated with diverse cellular activities (AAA)-type ATPase superfamily and consists of an N-terminal microtubule interacting and trafficking domain and a C-terminal AAA ATPase domain, connected by a disordered linker region. Microtubule promotes hexamerisation of the catalytic subunit, and stimulates ATPase and severing activities. Katanin hexamer forms a flat spiral ring, and pulls the tubulin C-terminal tail into the central pore. The electronegative multiple glutamates in the human β-tubulin are critical for binding and severing of microtubules (Zehr et al., Reference Zehr, Szyk, Szczesna and Roll-Mecak2020); plant β-tubulins are also rich in glutamates at their C-terminal tails. Microtubule is thought to break in the middle when sufficient numbers of tubulin heterodimers have been removed. Live imaging of Arabidopsis leaf epidermal cells suggests that several katanin hexamers need to accumulate at the severing sites before microtubule breakage occurs (Yagi et al., Reference Yagi, Kato, Matsunaga, Ehrhardt, Nakamura and Hashimoto2021).
Unlike mammals, which possess more than one katanin p60 genes (Lynn et al., Reference Lynn, Martinez, Nguyen and Torres2021), a single p60 gene is found in the Arabidopsis genome. Several genetic screens targeted for distinct phenotypes resulted in various allele names for Arabidopsis katanin mutants (Luptovčiak et al., Reference Luptovčiak, Komis, Takáč, Ovecka and Samaj2017). The p60 katanin mutants display disorganisation of cortical microtubule arrays and are defective in array reorganisation upon stimuli, which result in aberrant deposition of cellulose microfibrils, decreased cell wall strength, reduction in anisotropic cell expansion and a local decrease in growth heterogeneity (Bichet et al., Reference Bichet, Desnos, Turner, Grandjean and Höfte2001; Bouquin et al., Reference Bouquin, Mattsson, Naested, Foster and Mundy2003; Burk et al., Reference Burk, Liu, Zhong, Morrison and Ye2001; Lindeboom et al., Reference Lindeboom, Nakamura, Hibbel, Shundyak, Gutierrez, Ketelaar, Emons, Mulder, Kirik and Ehrhardt2013; Sassi et al., Reference Sassi, Ali, Boudon, Cloarec, Abad, Cellier, Chen, Gilles, Milani, Friml, Vernoux, Godin, Hamant and Traas2014; Uyttewaal et al., Reference Uyttewaal, Burian, Alim, Landrein, Borowska-Wykręt, Dedieu, Peaucelle, Ludynia, Traas, Boudaoud, Kwiatkowska and Hamant2012). Genetic screening also revealed that katanin-mediated microtubule dynamics plays a role in miRNA-guided translational repression (Brodersen et al., Reference Brodersen, Sakvarelidze-Achard, Bruun-Rasmussen, Dunoyer, Yamamoto, Sieburth and Voinnet2008).
The WD-40 repeat-containing p80 regulatory subunit promotes formation of a stable heterodimeric complex (Zehr et al., Reference Zehr, Szyk, Piszczek, Szczesna, Zuo and Roll-Mecak2017), and is essential for targeting of katanin to specific cellular severing sites, such as centrosomes (Hartman et al., Reference Hartman, Mahr, McNally, Okawa, Iwamatsu, Thomas, Cheesman, Heuser, Vale and McNally1998). The interface formed by p60 and p80 is recognised by animal katanin regulators that recruit katanin to the growing minus ends of microtubules (Jiang et al., Reference Jiang, Rezabkova, Hua, Liu, Capitani, Altelaar, Heck, Kammerer, Steinmetz and Akhmanova2017; Jiang et al., Reference Jiang, Faltova, Hua, Capitani, Prota, Landgraf, Volkmer, Kammerer, Steinmetz and Akhmanova2018). In Arabidopsis leaf epidermal cells of the p60 catalytic mutant, the p80 subunit by itself is still targeted to the normal subcellular severing sites, indicating that the regulatory subunit mediates subcellular targeting (Wang et al., Reference Wang, Liu, Wang, Li, Dong, Han, Wang, Tian, Yu, Gao and Kong2017). Arabidopsis possesses four p80 regulatory subunit genes. The quadruple p80 mutant shows growth and morphological phenotypes reminiscent of the p60 mutants (Wang et al., Reference Wang, Liu, Wang, Li, Dong, Han, Wang, Tian, Yu, Gao and Kong2017). Although they are largely redundant, it is possible that these subunits confer partly distinct regulatory roles to the p60–p80 complex in plant cells.
Recombinant Arabidopsis p60 subunit fragments Taxol-stabilised microtubules into shorter lengths (e.g., Burk & Ye, Reference Burk and Ye2002). Its overexpression in Arabidopsis causes similar fragmentation of cortical microtubules (Stoppin-Mellet et al., Reference Stoppin-Mellet, Gaillard and Vantard2006), suggesting that under such conditions, katanin severs microtubules along their length. However, in wild-type plant cells, katanin seldom severs microtubules along the entire lattice, but instead is recruited to select subcellular locations, owing to the regulatory functions of the p80 subunit (Wang et al., Reference Wang, Liu, Wang, Li, Dong, Han, Wang, Tian, Yu, Gao and Kong2017). Lateral cross-linking of adjacent microtubules by the microtubule-bundling protein MAP65-1 inhibits the binding of katanin along the sidewalls of microtubules in vitro (Burkart & Dixit, Reference Burkart and Dixit2019). The bundled microtubules may thus be inefficient substrates for katanin-mediated severing in plant cells. Major cellular severing sites by katanin are described below (Figure 1).
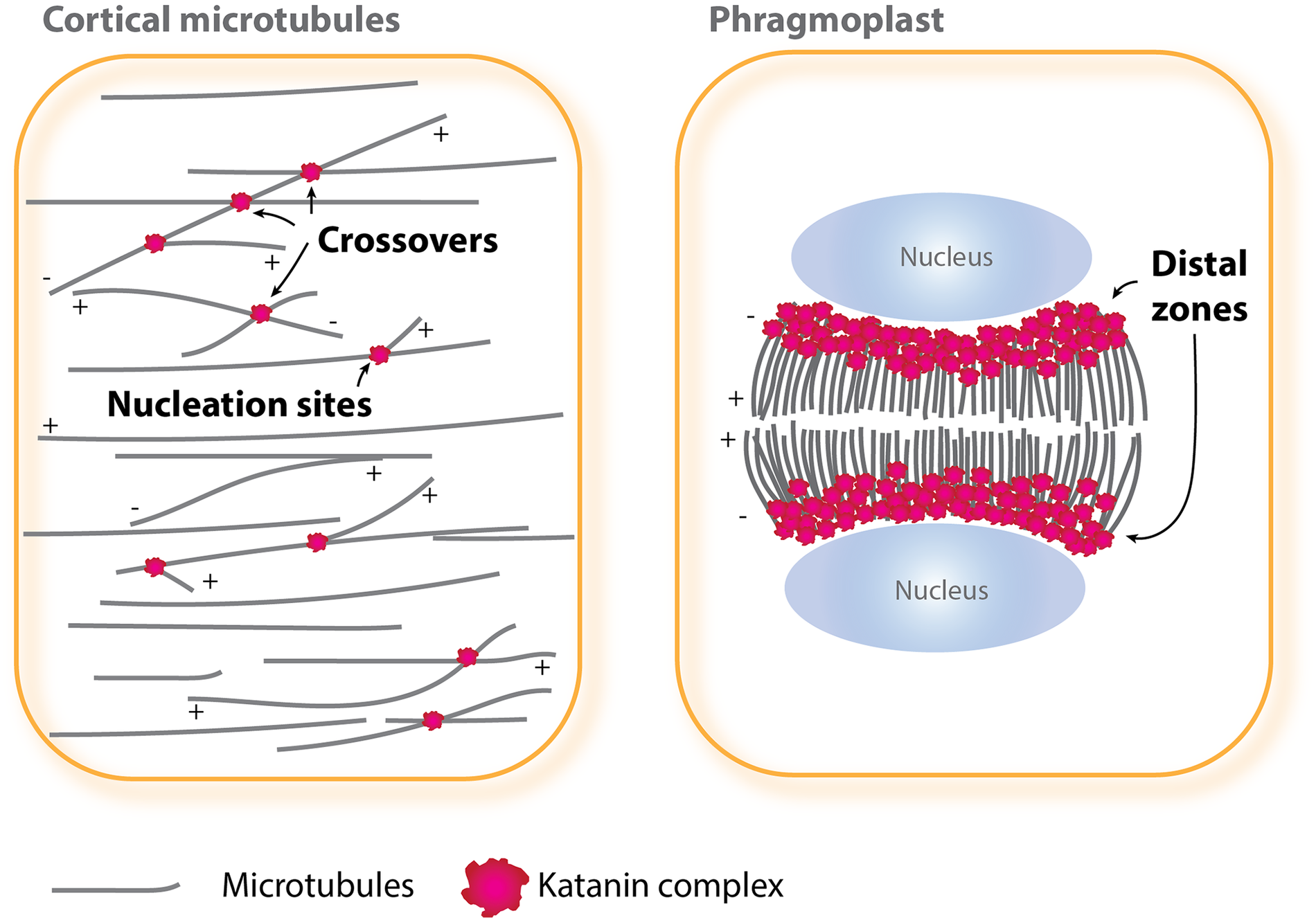
Fig. 1. Katanin-mediated microtubule severing in interphase and cytokinesis. In interphase cells (left), katanin is localised at and severs nucleation sites and crossover sites of cortical microtubules, whereas in cytokinetic cells (right), katanin functions at a distal zone of expanding phragmoplasts. The plus (+) and minus (−) ends of microtubules are indicated.
3. Crossover sites of interphase microtubules
In interphase plant cells, cortical microtubules are associated with the inner surface of the plasma membrane along their length, and migrate the cell cortex by a hybrid treadmilling mechanism (Shaw et al., Reference Shaw, Kamyar and Ehrhardt2003). Such dynamic microtubules frequently collide each other, thereby promoting ordering of the cortical array (Dixit & Cyr, Reference Dixit and Cyr2004). At the microtubule crossover sites, katanin accumulates and severs the crossing (or overlying) microtubules, rather than the crossed (or underlying) ones (Lindeboom et al., Reference Lindeboom, Nakamura, Hibbel, Shundyak, Gutierrez, Ketelaar, Emons, Mulder, Kirik and Ehrhardt2013; Wightman & Turner, Reference Wightman and Turner2007; Zhang et al., Reference Zhang, Fishel, Bertroche and Dixit2013). New plus ends of severed microtubules shrink rapidly in vitro (Walker et al., Reference Walker, Inoue and Salmon1989). In Arabidopsis hypocotyl epidermal cells, the lagging halves of the severed microtubules are largely depolymerised from the exposed plus ends (Zhang et al., Reference Zhang, Fishel, Bertroche and Dixit2013). However, upon illumination with blue light, new plus ends of severed microtubules are protected from depolymerisation, largely by the action of plus-end stabilising protein CLASP, and resume growing (Lindeboom et al., Reference Lindeboom, Nakamura, Saltini, Hibbel, Walia, Ketelaar, Emons, Sedbrook, Kirik, Mulder and Ehrhardt2019). Regrowth of severed microtubules amplifies the number of discordant microtubules and eventually reorients whole microtubule arrays to a new direction, which promotes curvature in response to light stimulus (Lindeboom et al., Reference Lindeboom, Nakamura, Hibbel, Shundyak, Gutierrez, Ketelaar, Emons, Mulder, Kirik and Ehrhardt2013). Katanin-mediated severing events also increase microtubule mass in non-plant organisms, such as at the meiotic spindle in Caenorhabditis elegans (Srayko et al., Reference Srayko, O’toole, Hyman and Müller-Reichert2006), indicating microtubule amplification by severing may be used in non-centrosomal microtubule arrays (Roll-Mecak & Vale, Reference Roll-Mecak and Vale2006). In silico simulations indicate that preferential severing of crossing microtubules at crossovers strongly enhances array alignment (Deinum et al., Reference Deinum, Tindemans, Lindeboom and Mulder2017).
How katanin recognises and is recruited to the microtubule crossovers? Short stretches of GTP -bound tubulins form a protecting GTP cap at the growing plus end of microtubules, while the main body of the microtubule shaft is composed of GDP-tubulins (Mitchison & Kirschner, Reference Mitchison and Kirschner1984). When dynamic microtubules were attached on coverslips and were set to collide each other in vitro, as observed for plant cortical microtubules, small GTP-tubulin patches accumulated at microtubule crossovers (de Forges et al., Reference de Forges, Pilon, Cantaloube, Pallandre, Haghiri-Gosnet, Perez and Poüs2016). In animal PtK2 cells, free tubulins (presumably in a GTP-bound form) were found to be incorporated to the microtubule lattice preferentially at crossovers (Aumeier et al., Reference Aumeier, Schaedel, Gaillard, John, Blanchoin and Théry2016). Free tubulin incorporation along the shafts of microtubules results from local microtubule damage and subsequent repair (Schaedel et al., Reference Schaedel, John, Gaillard, Nachury, Blanchoin and Théry2015). Since katanin has a strong affinity toward sites of damaged microtubule lattice in vitro (Davis et al., Reference Davis, Odde and Gross2002; Diaz-Valencia et al., Reference Diaz-Valencia, Morelli, Bailey, Zhang, Sharp and Ross2011), microtubule crossovers may be prone to local defects caused by geometric constraints and likely provide intrinsically high-affinity katanin binding sites (Figure 2). In the quasi-2D plane of plant cell cortex, crossing microtubules may experience more severe lattice deformation than crossed microtubules, thus leading to higher severing frequencies. Although local lattice defects intrinsically promote katanin recruitment at crossovers, this does not exclude elusive involvement of specific katanin targeting factors at these sites.

Fig. 2. Katanin-mediated microtubule severing at microtubule crossover sites. In plant interphase cells, crossovers are generated when two plasma membrane-associated cortical microtubules collide. Katanin accumulates at the crossover site by recognising elusive marks, possibly involving lattice defects, and severs an overriding microtubule. Since the precise geometry of microtubule crossover sites has not been clarified at the nanoscale level, the presented images are speculative and serve only for mechanistic discussions. The plus (+) and minus (−) ends of microtubules are indicated.
Microtubule severing probability at crossovers is influenced by factors other than katanin activities. SPIRAL2 is a minus-end tracking and stabilising protein (Fan et al., Reference Fan, Burkart and Dixit2018; Leong et al., Reference Leong, Yamada, Yanagisawa and Goshima2018; Nakamura et al., Reference Nakamura, Lindeboom, Saltini, Mulder and Ehrhardt2018). Suppressed minus-end depolymerisation of crossing microtubules increases lifetime of microtubule crossovers, resulting in a greater opportunity time for severing by katanin (Fan et al., Reference Fan, Burkart and Dixit2018; Nakamura et al., Reference Nakamura, Lindeboom, Saltini, Mulder and Ehrhardt2018). Although SPIRAL2 forms dynamic aggregates at crossovers, it remains controversial whether this microtubule regulator directly affects severing (Nakamura et al., Reference Nakamura, Lindeboom, Saltini, Mulder and Ehrhardt2018; Wightman et al., Reference Wightman, Chomicki, Kumar, Carr and Turner2013). A fraction of AUG3, a conserved subunit of augmin (see below), is also localised at microtubule crossovers (Wang et al., Reference Wang, Wang, Liu, Ma, Dong, Tian, Yu and Kong2018), but its potential roles there need further investigation.
4. Nucleation sites of interphase microtubules
Microtubules in eukaryotic cells are generally nucleated from ~2.2 MDa γ-tubulin complexes composed of γ-tubulin, related γ-tubulin complex proteins and a few other proteins (Thawani & Petry, Reference Thawani and Petry2021). The eight-subunit protein complex augmin recruits γ-tubulin complexes to the microtubule lattice to initiate microtubule-dependent microtubule nucleation (Song et al., Reference Song, King, Zhang, Kadzik, Thawani and Petry2018). In acentrosomal plant cells, microtubules are nucleated from γ-tubulin complexes mainly dispersed on the lattice of preexisting microtubules (Ehrhardt & Shaw, Reference Ehrhardt and Shaw2006; Murata et al., Reference Murata, Sonobe, Baskin, Hyodo, Hasezawa, Nagata, Horio and Hasebe2005; Nakamura et al., Reference Nakamura, Ehrhardt and Hashimoto2010) in an augmin-dependent manner (Lee et al., Reference Lee, Hiwatashi, Hotta, Xie, Doonan and Liu2017; Liu et al., Reference Liu, Tian, Wang, Yu, Wang, Ma, Zhang, Xia, Liu and Kong2014).
In interphase cortical arrays, microtubule-localised γ-tubulin complexes generate new (daughter) microtubules either at a branching angle of ~40° or in a parallel fashion, which results in immediate bundling with the mother microtubules (Chan et al., Reference Chan, Sambade, Calder and Lloyd2009; Murata et al., Reference Murata, Sonobe, Baskin, Hyodo, Hasezawa, Nagata, Horio and Hasebe2005; Yagi et al., Reference Yagi, Matsunaga and Hashimoto2018). The daughter microtubules are subsequently detached at the nucleation sites by katanin activities, and this nucleation-and-severing process is crucial for cortical microtubule array organisation and reorientation (Lindeboom et al., Reference Lindeboom, Nakamura, Hibbel, Shundyak, Gutierrez, Ketelaar, Emons, Mulder, Kirik and Ehrhardt2013; Zhang et al., Reference Zhang, Fishel, Bertroche and Dixit2013). In katanin mutant cells, daughter microtubules are not released from the nucleation sites and the γ-tubulin complexes remain attached at the nucleation sites (Nakamura et al., Reference Nakamura, Ehrhardt and Hashimoto2010). Katanin-mediated severing thus recycles once nucleated γ-tubulin complexes to a cytoplasmic pool for another round of nucleation.
Katanin is recruited to cortical microtubule nucleation sites by an evolutionally conserved tethering complex composed of mitotic spindle disanchored 1 (Msd1) and WD repeat-containing protein 8 (Wdr8). The Msd1–Wdr8 complex was initially discovered as a critical factor that anchors microtubule minus ends to spindle pole bodies, the centrosome equivalent in fission yeast, partly by interaction with γ-tubulin complexes (Toya et al., Reference Toya, Sato, Haselmann, Asakawa, Brunner, Antony and Toda2007; Yukawa et al., Reference Yukawa, Ikebe and Toda2015). In vertebrate cells, this complex tethers microtubules to the centrosome (Hori et al., Reference Hori, Peddie, Collinson and Toda2015). In Arabidopsis, Msd1 was first identified as a microtubule-associated protein (MAP), whereas Wdr8 was efficiently recovered in the MAP fractions but does not itself bind microtubules (Hamada et al., Reference Hamada2013). Recently, these two proteins were found to form complexes in vivo, and to be recruited to the cortical microtubule nucleation sites immediately following recruitment of γ-tubulin complexes there (Yagi et al., Reference Yagi, Kato, Matsunaga, Ehrhardt, Nakamura and Hashimoto2021; Figure 3). The complex strongly stabilises the branching nucleation sites; its absence leads to facile dissociation of the minus ends of daughter microtubules even without katanin activities. Remarkably, katanin recruitment to the cortical microtubule nucleation sites absolutely requires the Msd1–Wdr8 complex at these locations. Katanin recruitment to microtubule crossovers (see above) does not depend on this tethering complex, however. Thus, the plant Msd1–Wdr8 complex possesses dual functions; it tethers the minus ends of daughter microtubules to the nucleated γ-tubulin complexes on the mother microtubules, thereby stabilising the branched microtubule structures, while it also provides a katanin-recruitment site for subsequent severing and release of daughter microtubules. Such antagonising functions of Msd1–Wdr8 are thought to place the release of daughter microtubules under the strict control of katanin activities.

Fig. 3. Katanin-mediated severing and release of a daughter microtubule after nucleation. In plant interphase cells, both the γ-tubulin complex and the augmin complex accumulate at the lattice of existing cortical microtubules, and nucleate a daughter microtubule. After nucleation, the Msd1–Wdr8 complex is required to stabilise the branching nucleation structure; its absence results in spontaneous detachment of a daughter microtubule (yellow panel). The Msd1–Wdr8 stabilising complex subsequently recruits katanin for regulated severing and release of a daughter microtubule. The plus (+) and minus (−) ends of microtubules are indicated.
5. Microtubules at cell division
Mitotic spindle and the cytokinetic phragmoplast are bi-polar microtubule assemblies in which abundant microtubules are aligned in a roughly parallel fashion with their plus ends facing toward the midzone. Microtubule-dependent microtubule nucleation occurs in these arrays (e.g., Murata et al., Reference Murata, Sano, Sasabe, Nonaka, Higashiyama, Hasezawa, Machida and Hasebe2013) and requires γ-tubulin complexes and augmin (Lee & Liu, Reference Lee and Liu2019), but high microtubule densities make detailed cell biological analyses on nucleation and release of daughter microtubules challenging. Microtubule nucleation may be regulated differentially between interphase and mitotic cells, as suggested by distinct subunit compositions of augmin in interphase and mitosis (Lee et al., Reference Lee, Hiwatashi, Hotta, Xie, Doonan and Liu2017).
Katanin localises to mitotic microtubules arrays. Loss-of-function mutants of katanin p60 display misorientation of the mitotic plane (Bichet et al., Reference Bichet, Desnos, Turner, Grandjean and Höfte2001; Panteris et al., Reference Panteris, Adamakis, Voulgari and Papadopoulou2011; Webb et al., Reference Webb, Jouannic, Foreman, Linstead and Dolan2002), a delay in spindle positioning, and prolonged mitotic duration (Komis et al., Reference Komis, Luptovčiak, Ovecka, Samakovli, Samajova and Samaj2017). The shape of the phragmoplast is affected, and centrifugal expansion of phragmoplast is delayed (Komis et al., Reference Komis, Luptovčiak, Ovecka, Samakovli, Samajova and Samaj2017; Panteris et al., Reference Panteris, Adamakis, Voulgari and Papadopoulou2011). Whether katanin localises to nucleation sites, crossover sites or other sites of mitotic microtubules has not been critically determined due to dense array organisation. Subcellular localisation of Wdr8 largely overlaps with that of katanin on mitotic microtubules, but the Msd1–Wdr8 complex is not required for mitotic microtubule functions (Yagi et al., Reference Yagi, Kato, Matsunaga, Ehrhardt, Nakamura and Hashimoto2021), indicative of distinct regulation of katanin recruitment in mitotic cells. CORTICAL MICROTUBULE DISORDERING 4 (CORD4) and CORD5 are mitosis-expressed members of plant-specific MAPs (Sasaki et al., Reference Sasaki, Tsutsumi, Otomo, Murata, Yagi, Nakamura, Nemoto, Hasebe and Oda2019). These CORD proteins accumulate and co-localise with katanin at the distal phragmoplast zone. In the cord4 and cord5 mutant cells, katanin localisation expands to entire phragmoplast microtubules, which results in abnormally long and oblique phragmoplast microtubules and slow expansion of phragmoplasts. Ectopic expression of CORD4 in interphase plant cells caused recruitment of the katanin p80 regulatory subunit to cortical microtubules and their fragmentation in a katanin-dependent manner (Sasaki et al., Reference Sasaki, Tsutsumi, Otomo, Murata, Yagi, Nakamura, Nemoto, Hasebe and Oda2019). These results suggest that mitotic CORD proteins target katanin to the distal phragmoplast location (Figure 1), thereby promoting phragmoplast expansion by localised microtubule severing.
After cytokinesis, microtubules are temporally nucleated from the edge of newly formed cell walls, and the surface of nuclear envelope (Fishel & Dixit, Reference Fishel and Dixit2013). Although these microtubules may be released and incorporated into reorganised arrays, it is not known whether katanin is involved in these processes.
6. Outlook
Cellular katanin functions can be regulated by multiple levels, such as protein abundance, cellular localisation, microtubule binding and microtubule-severing activity. Blue light-stimulated and phototropin-dependent microtubule severing in plant cells (see above; Lindeboom et al., Reference Lindeboom, Nakamura, Hibbel, Shundyak, Gutierrez, Ketelaar, Emons, Mulder, Kirik and Ehrhardt2013) is an excellent example of environmental control of katanin functions. Studies in animal systems reveal that phosphorylation and ubiquitylation of katanin are particularly important regulatory mechanisms (Lynn et al., Reference Lynn, Martinez, Nguyen and Torres2021). Since katanin hexamers assembled in vitro from recombinant p60 subunit show robust severing activities, phosphorylation may generally function as inhibitory modifications. The katanin hexamer structure complexed with a tubulin C-terminus-mimicking peptide provides structural insight into how some phosphorylated katanin residues inhibit ATPase activity of katanin (Zehr et al., Reference Zehr, Szyk, Szczesna and Roll-Mecak2020). Several phosphorylation sites have been reported for plant katanin (e.g., Plant PTM Viewer; https://www.psb.ugent.be/webtools/ptm-viewer/index.php). Functional consequences of these phosphorylation sites need to be addressed in the future. Rho GTPase signalling pathway is another candidate for katanin regulation (Lin et al., Reference Lin, Cao, Zhou, Zhu, Ehrhardt, Yang and Fu2013).
Animal tubulin tails are posttranslationally modified with multiple glutamates, which are gripped by the katanin central pore and enhance severing activity (Zehr et al., Reference Zehr, Szyk, Szczesna and Roll-Mecak2020). Thus, glutamylation levels of tubulins provide potential regulatory mechanisms for katanin activity. However, plant tubulins show no evidence of posttranslational modification by glutamylation (Hotta et al., Reference Hotta, Fujita, Uchimura, Noguchi, Demura, Muto and Hashimoto2016), making tubulin glutamylation an unlikely regulation for plant katanin.
In this review, we described katanin as an enzyme that severs a microtubule into two fragments or detaches a daughter microtubule from the nucleation site. Interestingly, a recent in vitro reconstitution study demonstrated that worm katanin can partially damage the microtubule shaft by extracting several molecules of tubulin heterodimers, and the nanoscale damage sites are actively repaired by incorporation of GTP-bound tubulins from the solution (Vemu et al., Reference Vemu, Szczesna, Zehr, Spector, Grigorieff, Deaconescu and Roll-Mecak2018). The repaired site containing GTP-tubulins, in contrast to surrounding GDP-tubulin regions, now served as a ‘rescue’ spot where a shrinking plus end stops and regrows. Whether katanin in vivo nibbles particular microtubule locations, such as crossovers, and promotes amplification of microtubule mass without severing remains to be studied in plant cells.
Conflicts of interest
The authors declare no conflict of interest.
Financial support
The Japan Society for the Promotion of Science (JSPS) grants 20K21424 to M.N., 18KK0195 to M.N. and T.H., and 20H03276 to T.H., and the Human Frontier Science Program to M.N. ITbM is supported by the World Premier International Research Center Initiative (WPI), Japan.
Authorship contributions
All authors participated in writing the text and preparing the figures.
Data availability statement
No new data or code is presented in this paper.
Comments
December 20, 2021
Dear Editor of Quantitative Plant Biology;
We are herewith submitting a review article, entitles “Finding a right place to cut: how katanin is targeted to cellular severing sites”. Katanin severs plant microtubules at several subcellular locations, and molecular mechanisms for the selective targeting are recently uncovered. We believe that it is the right time to review this topic, and to appeal to the plant cell biologists.
Sincerely,
Takashi Hashimoto and Masayoshi Nakamura
Corresponding authors