Introduction
Rainfall is important to cereal crop production, since drought can reduce grain yield and quality (Gooding et al., Reference Gooding, Ellis, Shewry and Schofield2003; Sivakumar et al., Reference Sivakumar, Das and Brunini2005). In general, rainfall during vegetative growth promotes high yield, but heavy rainfall during seed development and maturation provides poor seed quality (Thomson, Reference Thomson1979; Tu et al., Reference Tu, McDonnell and Dirks1988; Olivares et al., Reference Olivares, Johnston and Calderon2009).
Wet summers in cool temperate environments are generally associated with poor wheat (Triticum aestivum L.) quality, whether for establishing subsequent crops (MacKay, Reference MacKay and Roberts1972) or for bread-making purposes (Smith and Gooding, Reference Smith and Gooding1999). Indeed, an association between the North Atlantic Oscillation, wet cool UK summers, and poor wheat seed quality has been noted (Kettlewell et al., Reference Kettlewell, Stephenson, Atkinson and Hollins2003) – including reduced specific weight through alternate wetting and drying cycles causing grain wrinkling. Moreover, rainfall before harvest may make harvesting conditions difficult (Landau et al., Reference Landau, Mitchell, Barnett, Colls, Craigon and Payne2000).
Under very wet and cool production environments, maturing wheat seed can show gross evidence of damage from rainfall due to viviparous germination, usually with readily visible pre-harvest sprouting (Mitchell et al., Reference Mitchell, Armstrong, Black, Chapman and Hebblethwaite1980; King, Reference King1993; Thomason et al., Reference Thomason, Hughes, Parrish and Barbeau2009). During seed development and maturation, wheat seed quality, as assessed by subsequent air-dry seed survival period (i.e. longevity), has been shown to improve progressively, reaching maximum values some 2–3 weeks after mass maturity (end of the seed-filling period) (Ellis and Pieta-Filho, Reference Ellis and Pieta-Filho1992; Sanhewe et al., Reference Sanhewe, Ellis, Hong, Wheeler, Batts, Hadley and Morison1996). Similar conclusions have been drawn in other cereals (Pieta-Filho and Ellis, Reference Pieta-Filho and Ellis1991; Rao et al., Reference Rao, Appa Rao, Mengesha and Ellis1991; Ellis et al., Reference Ellis, Hong and Jackson1993). These studies have shown subsequent seed longevity to be a more sensitive measure of seed quality than ability to germinate.
Anthropogenic greenhouse gas emissions, including carbon dioxide, continue to rise and climates later this century will be warmer with more intense and more frequent extreme precipitation events (Intergovernmental Panel on Climate Change, 2014). Sanhewe et al. (Reference Sanhewe, Ellis, Hong, Wheeler, Batts, Hadley and Morison1996) showed a progressive, positive effect of temperature (within the range investigated) on the development of subsequent wheat seed longevity, but no effect of carbon dioxide concentration. Here, we applied simulated rain to wheat crops at different stages of seed development and maturation to test the null hypotheses of no effect of rainfall on subsequent seed longevity and no interaction with developmental stage. The literature on extreme rainfall events is clear-cut, particularly where damage in the field prevents harvest, where for example pre-germination in barley (Hordeum vulgare L.) is known to reduce subsequent longevity (Gualano et al., Reference Gualano, Del Fueyo and Benech-Arnold2014). This study focused on less extreme treatments which did not result in pre-harvest sprouting, initially in the field and later in a protected environment, given that if germination does not occur, then wetting treatments to mature seeds (i.e. after harvest) can be of benefit to seed survival (Villiers and Edgecumbe, Reference Villiers and Edgecumbe1975).
Materials and methods
Wheat (Triticum aestivum L.) cv. Tybalt (suitable for autumn or spring sowing) was grown in the field at the Crop Research Unit, Sonning, UK (51°30′N, 00°54′W) in each of 2007–2008 and 2009, with standard agronomy (muriate of potash applied at 90 kg K2O ha− 1 in autumn before sowing; ammonium nitrate applied to crops in spring at 100 kg N ha− 1), or in pots in a polythene tunnel house at the Plant Environment Laboratory, Shinfield, UK in 2010 (51°27′N, 00°56′W).
2008
Seeds were sown 4 cm deep at 300 seeds per m2 in rows 12 cm apart on 31 October 2007 in plots 1.9 m wide, 5 m long and 0.6 m apart. Two blocks (A and B) were used in the field investigations to provide replication; results are presented separately for each block to provide information on the repeatability of any responses detected. Each block comprised three plots: control, natural rain only; wetting 1, simulated rain applied on 8 July 2008 [17 DAA (days after 50% anthesis)]; wetting 2, 23 July (32 DAA). Rainfall was simulated by applying the equivalent of 30 mm from above with a watering can with rose attached: half was applied first; then, after a 30-min delay, the remainder. Wettings were made at 08.00–10.00 h to allow time for subsequent harvests and laboratory work.
In the control, the first harvest was on 2 July 2008 (11 DAA). Subsequent harvests (2–6) were at 17, 23, 30, 37 and 51 DAA (11 August). In wetting 1, the first harvest was on 8 July 2008 (17 DAA) immediately before wetting, and the second 3 h after wetting (nominally 17.13 DAA) with harvests 3–7 at 20, 23, 30, 37 and 51 DAA. For wetting 2, the first harvest was on 23 July 2008 (32 DAA) immediately before wetting, and the second 3 h after (nominally 32.13 DAA) with harvests 3–6 at 34, 37, 39 and 51 DAA.
2009
Seeds were sown 4 cm deep at 400 seeds per m2 on 16 March 2009 in two blocks (other details as above), with four treatments: control, natural rain only; wetting 1, simulated rain on 13 July 2009 (29 DAA); wetting 2, simulated rain on 28 July (44 DAA); wetting 3, simulated rain on 11 August (58 DAA).
In the control, the first harvest was on 29 June (15 DAA). Subsequent harvests 2–7 were at 22, 29, 36, 44, 57 and 68 DAA (21 August), respectively. For wetting 1, the first harvest was on 13 July (29 DAA), the second 2 h after wetting (nominally 29.08 DAA), with harvests 3–7 at 31, 36, 44, 57 and 68 DAA. For wetting 2, the first harvest was on 27 July (43 DAA) immediately before wetting, and later ones (2–5) 2 h after wetting (nominally 43.08 DAA), 46, 57 and 68 DAA. For wetting 3, harvests 1–4 were from 11 August (58 DAA) immediately before wetting, 2 h after wetting (nominally 58.08 DAA), 60 and 68 DAA.
2010
Seeds were sown 2 cm deep in pots (18 cm diameter, 3 litre volume) at the equivalent of 215 seeds m− 2 on 14 April 2010. The pots were adjacent to each other in the middle of a well-ventilated polythene tunnel house (31 × 8 m), i.e. not subject to rain and only slightly warmer than ambient (fan-assisted ventilation was provided to reduce solar gain). The growing medium comprised sterilized vermiculite, sand, gravel and compost in the ratio of 4:2:4:1, mixed with Osmocote (Osmocote Exact-Scotts, Everris International B.V., Geldermalsen, The Netherlands) slow-release granules (2 kg m− 3) (N:P2O5:K2O:MgO; 15:11:13:2). Pots were irrigated through an automated drip-feed system; once a day initially, later twice a day. The rainfall simulation was the equivalent of 25 mm rainfall, with two treatments applied late in seed maturation. Control plants were harvested at 55 (10 August), 56 (11 August) or 62 DAA (17 August). Wetting 1 was at 56 DAA, with seeds harvested 30 min after wetting (nominally 56.02 DAA) and 6 d later (62 DAA). The second treatment comprised two simulated rainfall events 1 d apart, each of 25 mm, at 55 and 56 DAA. The first harvest was 30 min after the first wetting (nominally 55.02 DAA), the second just before (nominally 55.98 DAA) and the third 30 min after (nominally 56.02 DAA) the second wetting, with a final harvest at 62 DAA.
Seed harvest
Each harvest comprised 60–80 ears from an area of < 0.5 m2 selected at random within each plot. Samples were then taken to the laboratory and seeds threshed out by hand; 100 seeds plus 1 g were withdrawn from each sample to estimate ability to germinate, seed moisture content and seed weight. The remaining seeds were dried to 10–14% moisture content (wet basis) in a drying cabinet (15–17°C with 12–15% relative humidity), and each sample stored hermetically at − 20°C until the determination of longevity.
Seed moisture content
Seed moisture contents were determined using the high-constant-temperature-oven method (International Seed Testing Association, 2011), the only variation being that 2 × 100 seed samples were used in place of 2 × 4 − 5 g samples. The two-stage method (International Seed Testing Association, 2011) was used for moist seeds.
Ability to germinate
Freshly harvested seeds and dried seeds ( < 15% moisture content) were tested for ability to germinate between moist, rolled paper towels (Kimberley Clark Professional 6803 HOSTESS, Natural, 24 × 35 cm, Greenham Sales, UK) in an incubator at 10°C for 28 d, initially. Seeds remaining ungerminated and fresh were then pricked and returned to the test until all seeds had either germinated or rotted. Radicle emergence was the criterion of germination.
Seed longevity in hermetic storage
Seeds withdrawn from − 20°C were kept sealed overnight at 20°C to warm before opening the packets. The moisture content of each sample was adjusted (to 15 ± 0.2%) by humidification above water at 20°C (for 2–24 h, depending on initial moisture content), then stored hermetically at 3–5°C for 5 d, and a sample was withdrawn for moisture content determination. For each seed lot, 9–10 samples of 100 seeds (depending upon total sample size) were sealed in separate laminated-aluminium-foil bags (Retort laminate, Moore and Buckle Ltd, St Helens, UK). These bags were then stored in an incubator maintained at 40°C ( ± 0.5°C). Samples were removed from experimental storage to test ability to germinate (as above) at regular intervals for up to 34 (2008 and 2009) or 42 d (2010). Seed survival curves were fitted to the observations by probit analysis using Genstat (13th edition, VSN International Ltd., Hemel Hempstead, UK), in which analyses the criterion of survival was the ability of a seed to produce a normal seedling (International Seed Testing Association, 2011), providing estimates of σ, K i and p 50 in accordance with the seed viability equation (Ellis and Roberts, Reference Ellis and Roberts1980):

where v is probit percentage viability after p days in storage, σ is the standard deviation of the frequency distribution of seed deaths in time (d), K i is a constant (theoretical probit percentage viability at start of experimental storage) and p 50 (the product of K i and σ) is the period (d) in storage for viability to decline to 50%.
Results
Average mean temperature and total rainfall during seed development and maturation in the field were 16.7°C and 107.8 mm (anthesis to last harvest, 1 July to 11 August 2008); and 16.8°C and 92.6 mm (25 June to 21 August 2009). Hence, mean temperatures were similar amongst years and developing seeds received only 15.2 mm more rain in 2008 than 2009. Heavy rains (>10 mm d− 1, total 64 mm) occurred during the 3 weeks after anthesis in 2008, coinciding with the first treatment, while heavy rain (17 mm) occurred 3 d before the third wetting in 2009 (Fig. 1). The crops were observed to reach 50% anthesis on 21 June 2008 and 14 June 2009, with the end of the seed-filling period, mass maturity, at about 21 DAA (2008) and 25 DAA (2009). In the polythene tunnel house, the mean temperature from 50% anthesis (16 June 2010) to the last harvest was 19.7°C, with extremes of 28.5 and 9.4°C. While the summer ambient temperature in 2010 was marginally warmer than either 2008 or 2009, the higher mean temperature in 2010 given above largely reflects the effect of the protected environment.
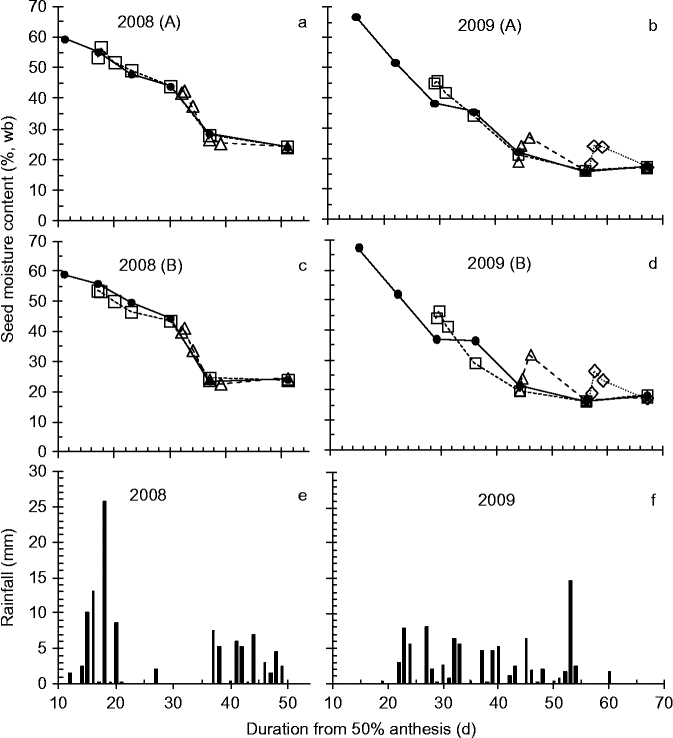
Figure 1 Effect of simulated rainfall (30 mm applied above the canopy) on the moisture content [%, wet basis (wb)] of wheat seeds harvested serially during development and maturation of field-grown crops for control (–●–, no simulated additional rainfall), wetting 1 (…□…), wetting 2 (- -△- -) or wetting 3 () treatments in 2008 (a, c) or 2009 (b, d) in Blocks A (a, b) and B (c, d), and the rainfall (mm) received each day during the experimental periods (e, f).
Seed moisture content
In the two field-crop studies, seed moisture content in the control declined more slowly in the 6 or so weeks after anthesis in 2008 than in 2009 – from 59% at 11 DAA to about 24% at 37 DAA in 2008 (Fig. 1a, c), and from 67% at 15 DAA to about 22% at 44 DAA in 2009 (Fig. 1b, d) – in accordance with differences in rainfall over this period between years (Fig. 1e, f).
In 2008, the two simulated rainfall treatments had no detectable effect (P > 0.25) on seed moisture content (Fig. 1a, c), whereas in 2009 all three treatments increased (P < 0.05) seed moisture content by some 6–10% temporarily, with control values regained some 5–10 d later (Fig. 1b, d). In 2010, seed moisture content of the control, at and after harvest maturity, was stable at 14.1–14.5% during the experimental period (Fig. 2). Immediately after simulated rainfall, seed moisture content increased (P < 0.05) by 4.3–5.0%, but reverted to control values as soon as 24 h later (Fig. 2).
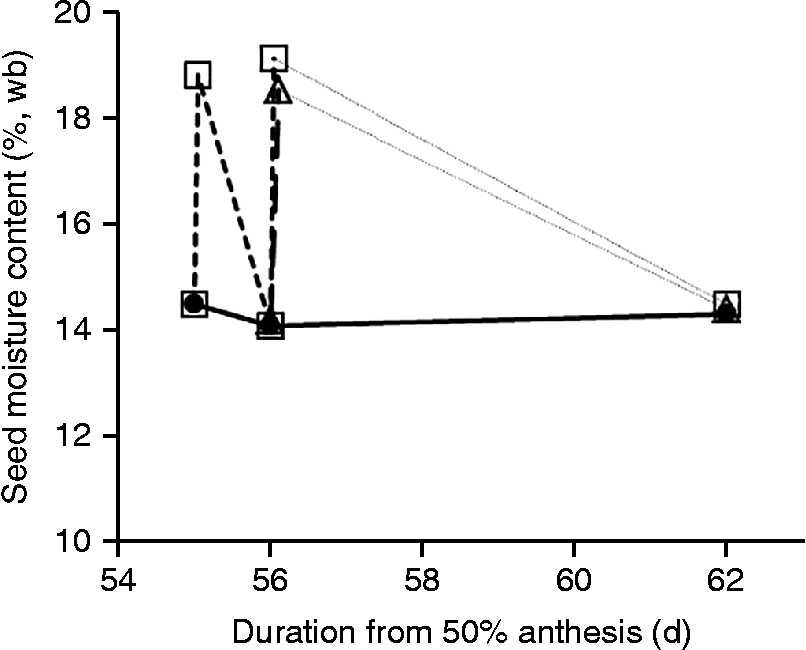
Figure 2 Effect of simulated rainfall (25 mm applied above the canopy) on the moisture content (%, wb) of wheat seeds from serial harvests at or after harvest maturity produced protected from rainfall in a polythene tunnel house for control (, no simulated rainfall), wetting once at 56 DAA (
) or wetting on two consecutive days (
) at 55 and 56 DAA. The dotted lines joining symbols between 56 and 62 DAA do not represent the probable negative exponential pattern of drying.
Seed viability and longevity
No pre-harvest sprouting occurred in the controls or treatments in any year. With dormancy overcome by testing at 10°C combined with pricking, the majority of seeds were able to germinate from the earliest harvests (e.g. 91% at early as 11 DAA in 2008), such that all samples from 23 DAA until the last harvests for all treatments showed 100%, or close to, viability (data not shown).
Within each of the 3 years, seed survival curves varied substantially (Table 1) amongst treatment combinations: K i varied significantly with wetting treatment (P < 0.001 for 2008, 2009 and 2010), harvest date (P < 0.001, P < 0.01, P < 0.001 for 2008, 2009 and 2010, respectively) and harvest date × treatment (P < 0.05, P < 0.001 for 2008 and 2009, respectively); and 1/σ varied significantly with wetting treatment (P < 0.05, P < 0.005, P < 0.001 for 2008, 2009 and 2010, respectively), harvest date (P < 0.001, P < 0.01, P < 0.001 for 2008, 2009 and 2010, respectively) and harvest date × treatment (P < 0.001 for 2008 and 2009). There was a 3.5-fold maximum variation in longevity (p 50) within 2008, 4.1-fold in 2009, and 2.7-fold in 2010, with differences in both K i and σ (Table 1). The major driver of this variation in the 2008 and 2009 field investigations was the period of seed development and maturation (Fig. 3). In contrast, in 2010, where samples were only harvested after the end of maturation drying, the variation in longevity resulted from wetting treatments (Fig. 4).
Table 1 Estimates of the seed lot constant (K i ), slope (1/σ) and longevity (p 50, d) for seed survival curves of wheat cv. Tybalt produced in 2008, 2009 and 2010 under different wetting regimes harvested at different times during seed development and maturation. Seed survival curves were fitted by probit analysis in accordance with the seed viability equation (Ellis and Roberts, Reference Ellis and Roberts1980)
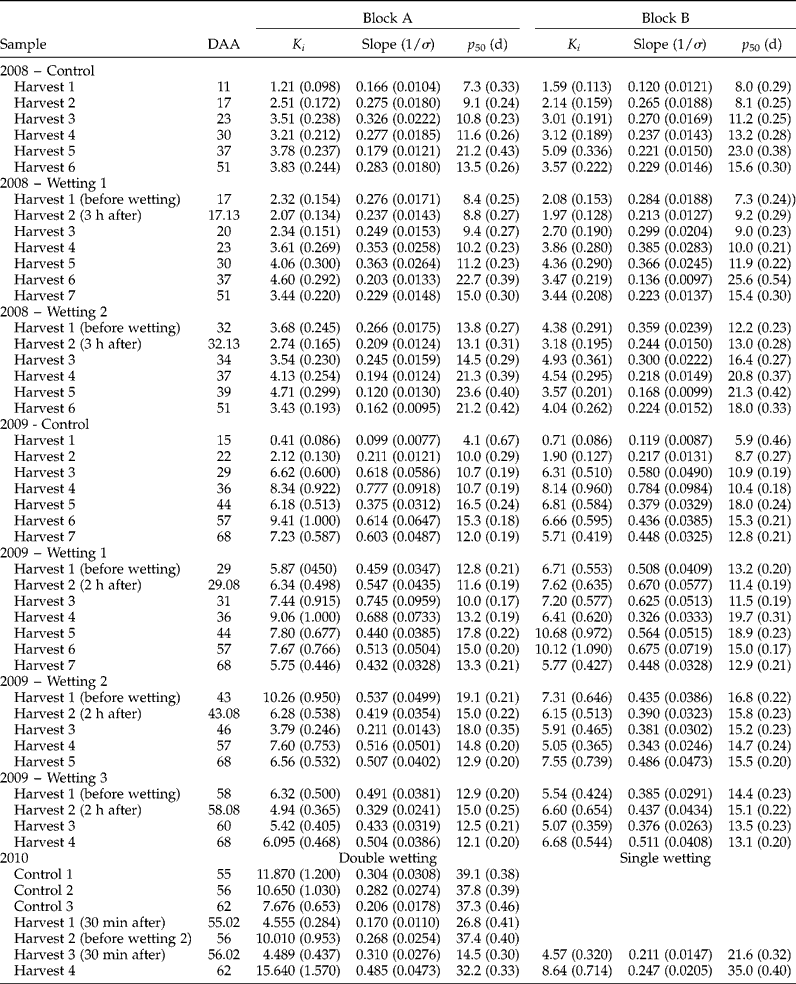
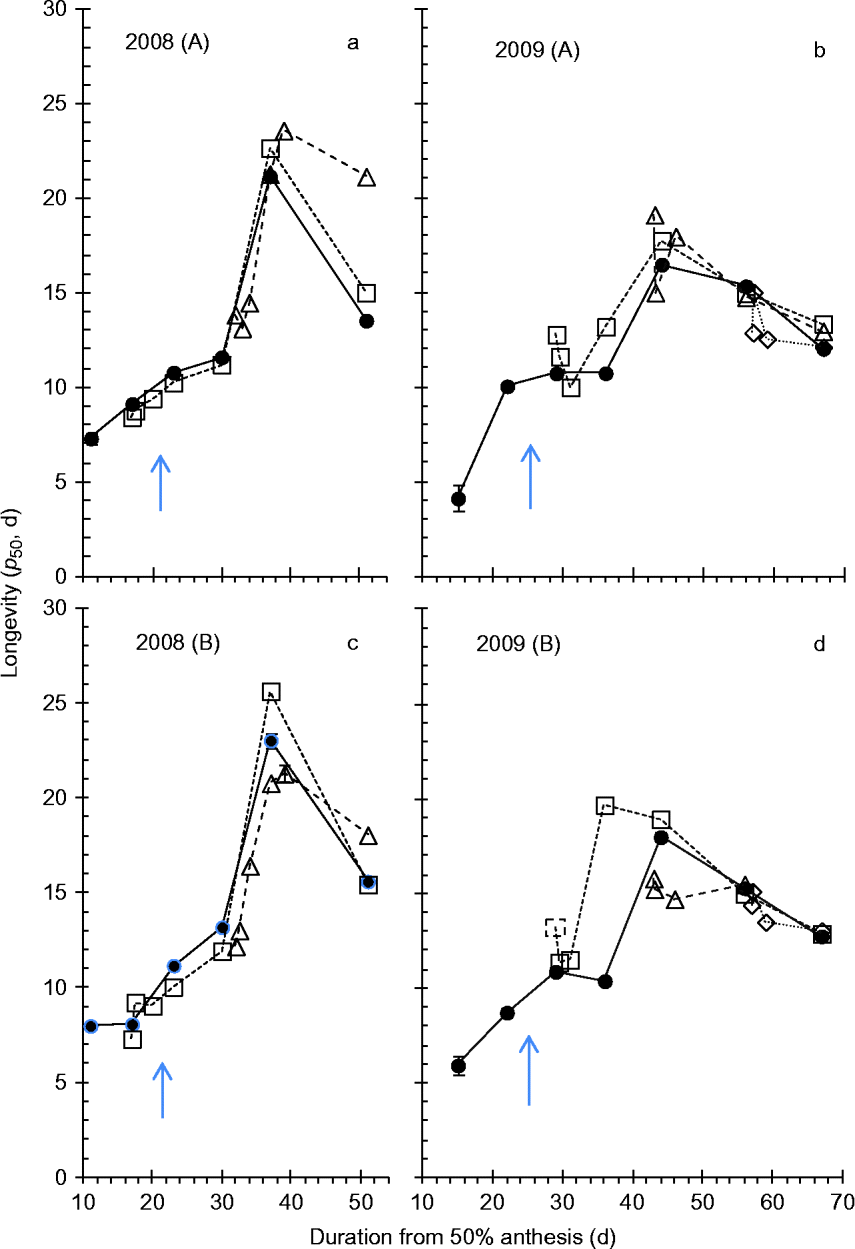
Figure 3 Effect of simulated rainfall (30 mm applied above the canopy) on the subsequent air-dry seed storage longevity (p
50, period of experimental hermetic storage at 40°C with c. 15% moisture content until viability reduced to 50%, estimates provided by probit analysis, Table 1) of wheat seeds harvested serially during their development and maturation from field-grown crops in Blocks A (a, b) and B (c, d) in 2008 (a, c) or 2009 (b, d) for the control (–●–, no simulated additional rainfall), wetting 1 (…□…) and wetting 2 (- -△- -) in 2008, or also with wetting 3 () in 2009. Vertical bars show standard errors of the estimates of p
50 where larger than symbols. Arrows indicate mass maturity.

Figure 4 Effect of simulated rainfall (25 mm applied above the canopy) on the subsequent air-dry seed storage longevity (p
50, period of experimental hermetic storage at 40°C with c. 15% moisture content until viability reduced to 50%, estimates provided by probit analysis, Table 1) of wheat seeds harvested serially at or after harvest maturity produced protected from rainfall in a polythene tunnel house in 2010 for control (, no simulated rainfall), wetting once at 56 DAA (
) or on two consecutive days (
) at 55 and 56 DAA. The dotted lines joining symbols between 56 and 62 DAA may not represent the real pattern. Vertical bars show standard errors of the estimates of p
50 where larger than symbols.
In both 2008 and 2009, clear and consistent (across both blocks and treatments) patterns of the effect of developmental period on longevity were detected, with increase in longevity until 37–39 DAA (2008) or 44–46 DAA (2009) with a subsequent decline in longevity thereafter (Fig. 3). There was a single outlier to the above: Block B wetting 1 in 2009, where peak longevity occurred earlier, at 36 DAA (Fig. 3d).
In 2008, wetting 1 at 17 DAA (about 4 d before mass maturity, when seed moisture content was about 55–56%) led to an almost consistent but small reduction in longevity compared with the control in the period 17–30 DAA (Fig. 3a, c). This was equivalent to a 2- to 4-d delay in the developmental pattern of improvement in longevity. From 37 DAA onwards, however, longevity was no less than the control and often slightly greater. Wetting 2 at 32 DAA (about 11 d after mass maturity, when seed moisture content was close to 40%), also led to slightly shorter longevity than the control over the subsequent 2 (Block A, Fig. 3a) to 5 d (Block B, Fig. 3c), equivalent to about a 4-d delay in the developmental pattern of improvement in longevity, but thereafter longevity was similar to, or slightly greater than, the control.
In 2009, wetting 1 at 29 DAA (4 d after mass maturity, when seed moisture content had declined to 37–38%) resulted in some fluctuation in longevity (a brief reduction on the day of wetting), but throughout subsequent maturation drying longevity was no less than the control (Fig. 3b, d). Wetting 2 at 43 DAA (18 d after mass maturity, when moisture content had declined to 21–23%) similarly provided a brief reduction in longevity, but with later harvests identical to the control. Wetting 3 applied at 58 DAA (33 d after mass maturity, when moisture content had declined to about 16%) similarly led to a brief reduction in longevity, but with later harvests identical to the control.
The longevity of the control in the protected environment of 2010 varied only from 39 d at 55 DAA to 37 d at 62 DAA, but all three wetting treatments reduced longevity immediately (P < 0.05) and substantially (Fig. 4). Subsequently, however, longevity improved to (first wetting at 55 DAA, P>0.10), or towards (remaining treatments at 62 DAA, P < 0.05), control values. Single wettings were less damaging to longevity than wetting on two consecutive days (P < 0.05).
Discussion
The pattern of increase in subsequent air-dry longevity during seed development and maturation in the field to maxima at around 16 (2008) to 21 d (2009) after mass maturity is in accordance with earlier studies in wheat (Ellis and Pieta-Filho, Reference Ellis and Pieta-Filho1992; Sanhewe et al., Reference Sanhewe, Ellis, Hong, Wheeler, Batts, Hadley and Morison1996). There was good agreement between the 2 years of field experiments for estimates of peak longevity in the controls (p 50= 17–23 d, Fig. 3), which received considerable rainfall (Fig. 1e, f), whereas the 2010 controls protected from rainfall in the polythene tunnel house provided substantially greater estimates (p 50= 37–39 d, Fig. 4). This investigation was not designed to enable comparisons amongst years but, while a benefit from protection from rainfall throughout seed development and maturation in 2010 cannot be ruled out, it seems probable from Sanhewe et al. (Reference Sanhewe, Ellis, Hong, Wheeler, Batts, Hadley and Morison1996) that the 3°C warmer environment is the more likely (though not necessarily exclusive) explanation.
Provided seed germination can be prevented, air-dry seed storage survival may, on occasion, benefit from wetting–drying cycles: for example, repeated imbibition–drying cycles to mature seed ex planta aided lettuce (Lactuca sativa L.) seed long-term survival (Villiers and Edgecumbe, Reference Villiers and Edgecumbe1975); and the air-dry longevity of foxglove (Digitalis purpurea L.) seeds harvested close to maturity could be increased by immediate priming and dehydration (Butler et al., Reference Butler, Hay, Ellis, Smith and Murray2009). The topic is controversial, however, since many studies of seed priming and other forms of rehydration have been shown to damage longevity (e.g. Argerich et al., Reference Argerich, Bradford and Tarquis1989; Tarquis and Bradford, Reference Tarquis and Bradford1992) and desiccation tolerance (Hong and Ellis, Reference Hong and Ellis1992).
Both wetting treatments to developing wheat seeds in 2008 were before peak longevity was detected. Immediate, small reductions in longevity resulted from wetting in these circumstances (essentially a 2- to 4-d perturbation which delayed the pattern of seed quality improvement), but there was no evidence of reduced longevity relative to the control in the later samples harvested after 37 DAA (Fig. 3a, c). Simulated rainfall was applied later in development in 2009, and the second and third treatments, at about the point of peak longevity or 15 d later, showed evidence of slight damage to longevity after wetting but, similarly, close agreement with control estimates amongst later harvests (Fig. 3b, d). In the protected environment of 2010, wetting only at or after harvest maturity, there was a far greater and more repeatable pattern of an immediate reduction in longevity after wetting with subsequent improvement towards estimates similar to the control (Fig. 4). Moreover, comparison with the third wetting treatment in 2009, the only field treatment applied close to harvest maturity, shows good agreement with the 2010 pattern. Provided that germination does not occur, we suggest that the reversible effect detected here in wheat could occur in other plant species with seeds that undergo maturation drying with little protection from rainfall.
Oligosaccharides (e.g. Crowe et al., Reference Crowe, Crowe and Chapman1984; Leopold, Reference Leopold, Alscher and Cumming1990) and low molecular weight heat-stable proteins (e.g. Galau et al., Reference Galau, Hughes and Dure1986) have been posited to play significant roles in seed survival in the air-dry state. Both have been associated with improvements to subsequent seed longevity during seed development and maturation, but with oligosaccharides accumulating more during development and heat-stable proteins more during maturation drying (Sinniah et al., Reference Sinniah, Ellis and John1998). Similarly, therefore, we would caution against the simple notion that the cause(s) of the immediate reduction to subsequent longevity and later reversal of damage is the same during the seed-filling phase as during maturation drying and/or at harvest maturity. In the latter case, the decline in longevity within a few hours of wetting and rapid subsequent recovery, in only 1 d, apparent at 55–56 DAA in 2010 (Fig. 4) at comparatively low moisture contents (Fig. 2), would seem to militate against, for example, complex carbohydrate chemistry or considerable protein synthesis (as occur substantially earlier in development). Wetting–drying cycles affect the degree of hysteresis of seed moisture content–equilibrium relative humidity isotherms in mature wheat seeds (Pixton and Warburton, Reference Pixton and Warburton1973). The formation of intracellular glasses is one of the factors reported to be indispensable to air-dry seed survival (Buitink and Leprince, Reference Buitink and Leprince2004). Cells compress during desiccation, with glass formation providing protection from such damage, and Walters (Reference Walters2015) has suggested that the resultant newly formed intra-molecular spatial arrangements affect seed survival. Such hypotheses may be relevant to the effects detected here at harvest maturity. Hence, the experimental approaches developed here might be of utility to those investigating the fundamental basis of seed survival in the air-dry state.
Research by Woodward (Reference Woodward1990) and Piggot and Huntley (Reference Pigott and Huntley1981), for example, has provided clear evidence of the importance of patterns of temperature at critical stages in seed formation and development to the geographic distribution of plant species. Similarly, rainfall patterns affect plant distribution greatly (Woodward, Reference Woodward1996). The effects shown here in wheat cv. Tybalt may have possible parallels in wild species. For example, in Galanthus nivalis and Narcissus pseudonarcissus, hydration in the summer period after shedding in late spring enabled further seed development and maturation, which affected subsequent patterns of loss in dormancy in response to seasonal patterns of temperature (Newton et al., Reference Newton, Hay and Ellis2013, Reference Newton, Hay and Ellis2015). Hence, if they occur in other species, then at a minimum such effects of rainfall on longevity may affect subsequent gap finding and competitive ability in seed–seedling cycles – by affecting not the amount but the quality of seeds produced – and potentially the geographic distribution of a species if such effects occur at the edges of their ranges, and potentially in response to climate change.
We caution that the novel effects reported here are for a single cultivar of one (crop) plant species. Within that limitation, we conclude that rainfall causing ear wetting in planta late in maturation drying, insufficient to result in pre-harvest sprouting, may nonetheless reduce subsequent ex planta air-dry seed longevity immediately, but that in such circumstances the damage can be reversed by delaying harvest to enable a period of re-drying in planta. In terms of seed production practices, this supports the traditional UK approach of delaying wheat seed harvest in wet conditions until the crop re-dries. The evidence that wetting during seed filling may also damage longevity is less clear but, in this case also, damage was reversed with further development in planta. Hence, we are not able to conclude whether damage from rainfall to subsequent seed longevity is subject to interaction with stage of seed development.
Acknowledgements
We thank Caroline Hadley, Richard Casebow, Laurence Hansen and Dr Tran Dang-Hong for their considerable help with this study.
Financial support
The Commonwealth Scholarship Commission provided a research studentship to G.Y.
Conflicts of interest
None.