An Introduction to String Multiphonics
I define a string multiphonic as a single excitation by which two or more harmonics sound distinctly and simultaneously on a touched string. I use the term ‘harmonics’ here rather than ‘partials’ because it fits my understanding of how multiphonics are perceived. It seems that the ear organises the components into groups of harmonics: for example, a multiphonic with partials 3, 5, 6, 8, 10, 12, 13, 15 can be reduced to [3, 5, 8, 13]Footnote 1 since 6 (2 × 3) and 12 (4 × 3) are octave multiples of the 3rd harmonic, 15 (5 × 3) is the 5th overtone of the 3rd harmonic/3rd overtone of the 5th harmonic, and 10 (2 × 5) is the octave multiple of the 5th harmonic. When I use ‘partial’ in this text, I am referring to individual partials separately from their harmonic relations.
In previous research, I derived a set of cello multiphonics by working at the instrument and testing each node of a subset of the first 13 harmonics on the string to try and induce harmonics with neighbouring nodes to sound together. This resulted in eight multiphonics, each with two left-hand fingering positions, shown in Figure 1.
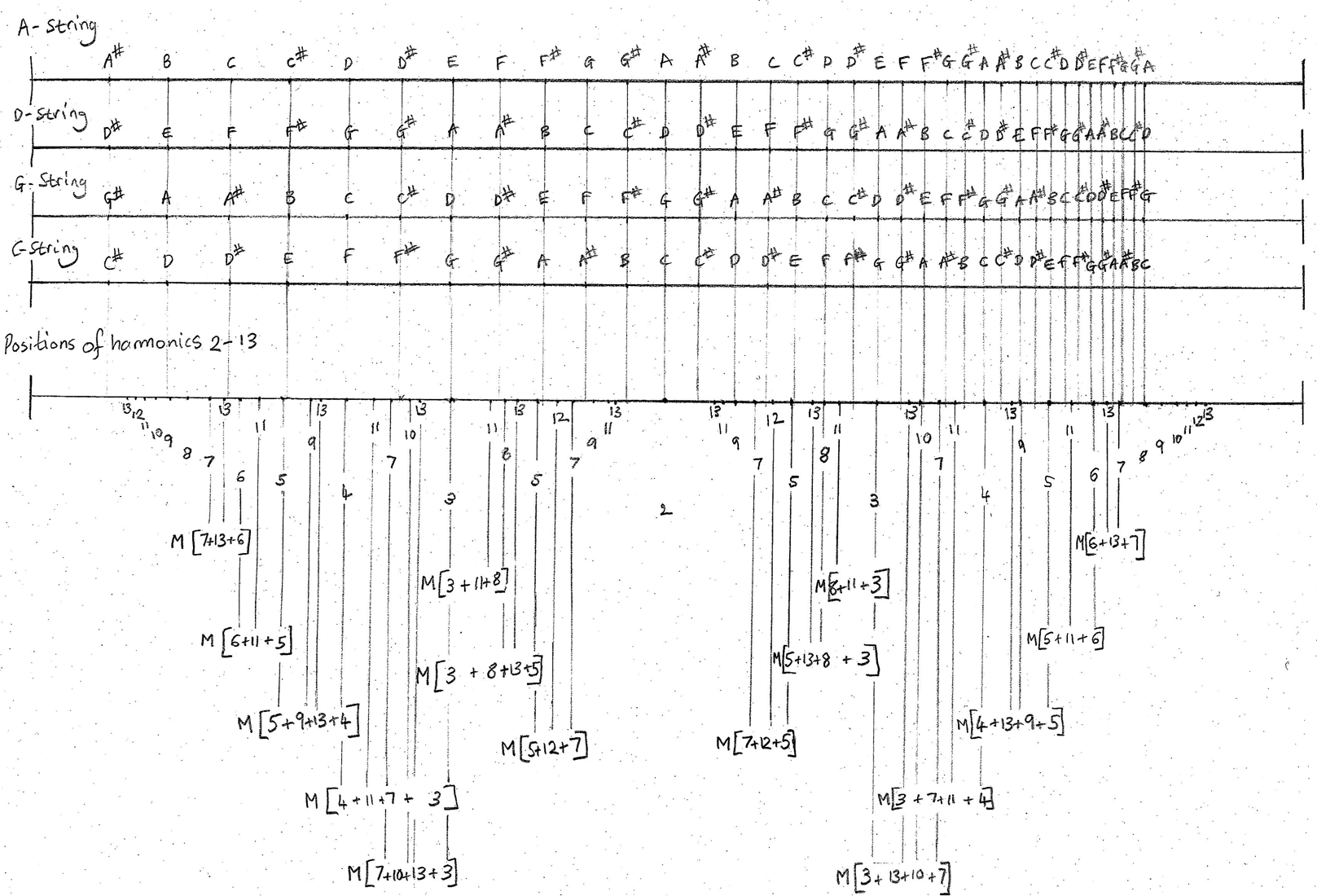
Figure 1: A sketch of a string showing nodal points of the first 13 harmonics and 8 multiphonics that contain these harmonics, taken from Cello Map.Footnote 2
It can be clearly seen in Figure 1 that, because the arrangement of harmonic nodes on the string is symmetrical, there are two equivalent playing positions for each multiphonic, equidistant from the string's midpoint. Taking the multiphonic fingering position as the nodal point of the highest contributing harmonic, I created fingering charts.Footnote 3
Caspar Johannes Walter's algorithm for predicting the harmonic components of multiphonics is an important addition to the literature because it tells musicians what to search for.Footnote 4 From this stable theoretical framework it is easier to identify other aspects of multiphonics, such as additional pitches that may be present. A glance at the multiphonic notations above suggests that mathematical relations exist: all harmonic numbers are either sums or differences of two others. Walter's algorithm concretizes this: he starts with the Fibonacci series (1, 2, 3, 5, 8, 13 …) and then makes mutations upon this series to create a potentially infinite set of multiphonic branches. Because this provides a theoretically infinite list of contributing harmonics, it is necessary to fix certain boundaries to decide which components make up a multiphonic in practice. These boundaries are different for each instrument (potentially even each string), and they vary according to playing methods and conditions (bowing vs plucking, hard vs soft plectrum, etc.). Producing a fingering chart for the piano, Walter decided to restrict multiphonic aggregates to sets of the four harmonic components that he considers the most audible. Figure 2 compares Walter's theoretical predictions with the results that instrumentalists have produced according to experimentation at the instrument, sometimes with the assistance of spectral analysis. The results slot together pleasingly well. Moreover, most instrumentalists restrict the multiphonics to groups of three or four tones, and almost all disregard the open string which, although usually audible, apparently does not seem to be considered a significant aspect of multiphonic sound (this will be discussed in more detail later).
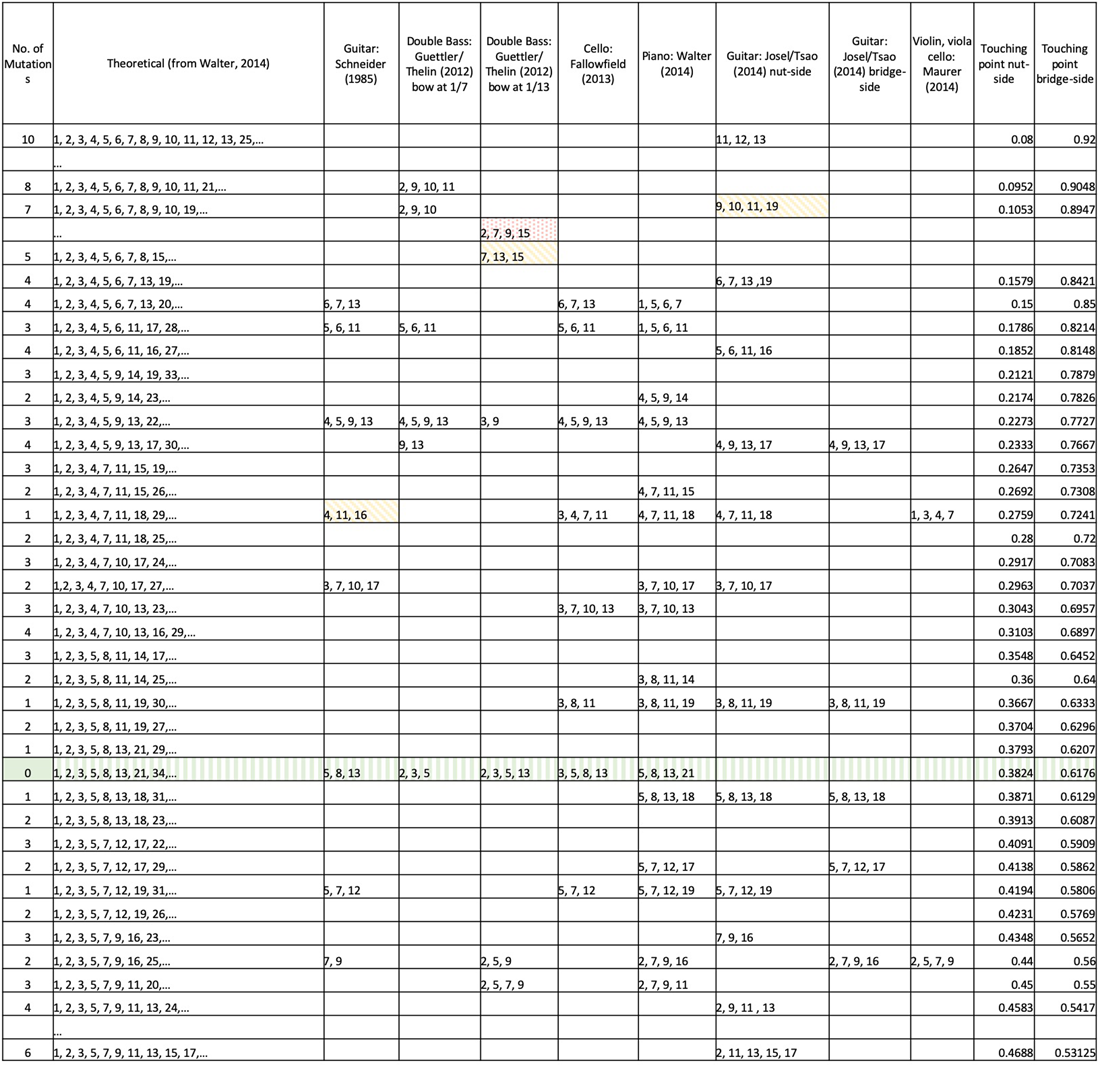
Figure 2: Chronological publication of multiphonic fingering charts for string players.
The column on the far left lists the number of mutations from the Fibonacci series according to Walter's algorithm. The ‘Fibonacci multiphonic’, with 0 mutations, is shaded in vertical stripes. The column second from the left shows theoretical outcomes of Walter's algorithm. The subsequent seven columns list the multiphonics found by instrumentalists chronologically according to their publication (Schneider, Guettler/Thelin, Fallowfield, Walter, Josel and Tsao, MaurerFootnote 5). The two right-hand columns express the left-hand fingering position (‘touching point’) as a proportion of string length (from top to bottom: nut to mid-point and bridge to mid-point, respectively), this was defined as the nodal point of the highest contributing harmonic. In the interests of space, I skipped some iterations of the algorithm that did not directly refer to published findings. This is indicated by ‘…’. Three results with one anomalous harmonic component are shaded in diagonal stripes, and the only result which cannot be slotted directly into Walter's theoretical model is shaded in dots.
Expanding upon the above alignment of theoretical and practical results, consider Knut Guettler's physical definition. Guettler and Thelin undertook the first (and thus far, only) detailed physical analysis of string multiphonics. According to Guettler: ‘In string instruments, multiphonics is mainly a filtering technique where the potential energy of certain partials of an (in most cases) open-string fundamental is restrained by a left-hand finger pad lightly touching the string. This favours the conditions for some of the remaining partials, separately or in narrow clusters.’Footnote 6 He goes on to say that the ‘filtering’ is not superimposed on an established waveform (cf. Helmholtz), but rather ‘defines the waves’ environment on the string’.Footnote 7 This description seems to me to open the possibility of pitches extra to the mathematical theoretical model that might be ‘filtered-in’ to the sound. The concept of narrow clusters is also important, and I will return to this later.
From Figure 2, the results that musicians have gravitated towards by using their instruments and ears (and sometimes spectral analysis) already give a sense of the superimposition of instruments’ technical limitations upon the theory. However, the empty spaces in the grid are inviting to curious and ambitious musicians and prompt the question: can we fill in the gaps? In other words, for cello multiphonics, can we use the theoretical model to expand on the possibilities set out in Cello Map, and where do we set the technical limits?
I approached this question by undertaking spectral analyses of cello multiphonics. I tried to interpret the results in the context of what is audible and not to draw conclusions only from analysis. There might seem to be a contradiction in comparing the subjective aural impression of a sound with an analytical snapshot of several milliseconds, but I have tried to achieve a balance and, where necessary, I have compared several samples from different phases of the envelope. I am aware that the number of data points and the conditions of empirical testing would need to be considerably expanded to meet those usually required for scientific analysis. However, I hope to elicit interest amongst colleagues in physics and acoustics who might want to take up these research topics. To ensure that I was not simply recording a ‘personalisation’ of technical special-effects, two cellist colleagues and I rotated three cellos between us, each cellist playing several multiphonics on each cello. I also had access to a monochord on which I recorded several plucked multiphonics. The long strings make this an interesting comparative model to the cello. Details about the recordings can be found in the appendix. As well as ‘de-personalising’ the playing technique I attempted to add an objective ear by undertaking a listening test on some of the recordings with a group of composition students at the Hochschule für Musik, Basel.
Pitch content
The pitch content of the cello multiphonics that I recorded lines up well with Walter's theoretical predictions. The majority of the highest-amplitude partials in the sound are those predicted by the algorithm or their octave multiples. For example, an analysis of three representative recordings are shown in Figure 3. Partials directly predicted by Walter's algorithm and their octave multiples are shaded (with the exception of the 2nd partial, to be discussed later) and octave multiples of these are marked in horizontal stripes. The five highest-amplitude partials all fall into one of these two categories.
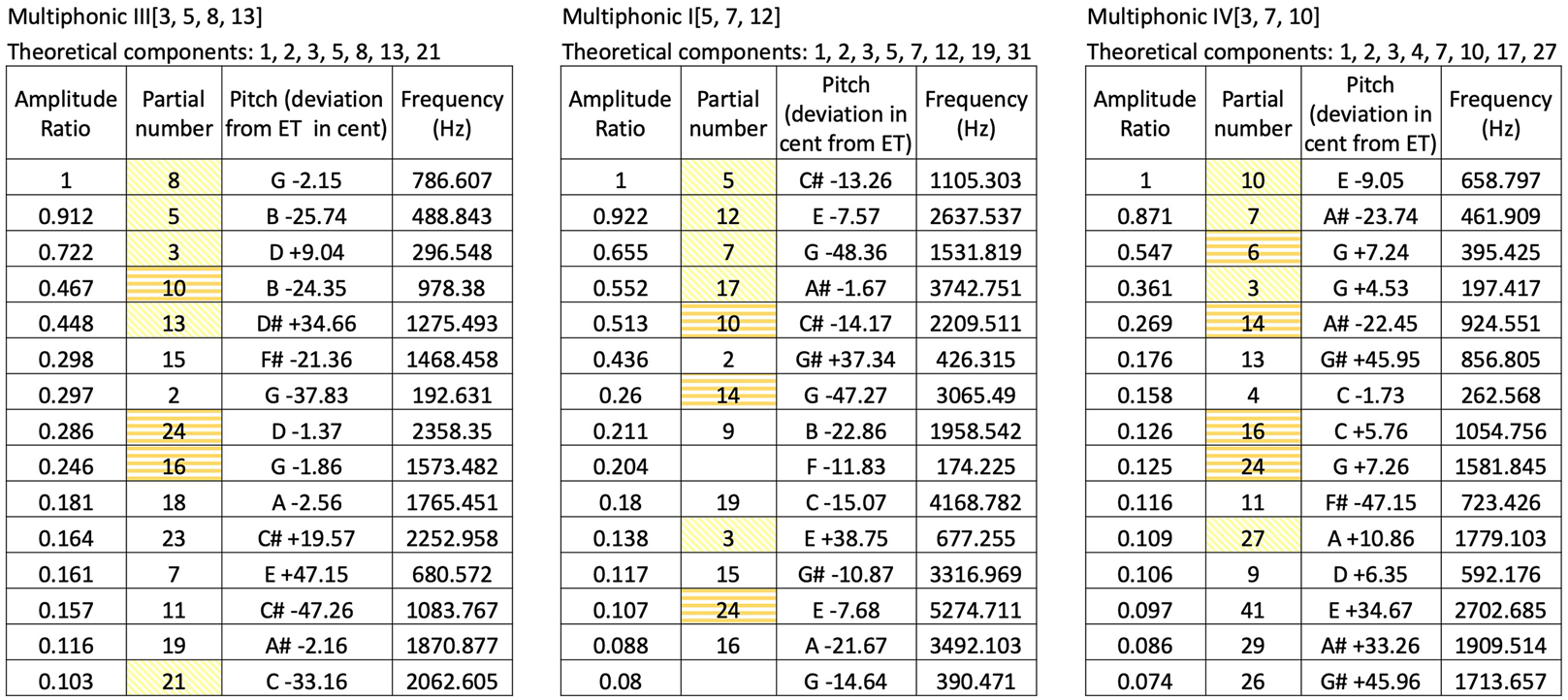
Figure 3: Pitch Content: Three representative Multiphonics performed on three cellos by three different cellists on three different strings (See Audio Examples 1–3).Footnote 8
The column on the far left of Figure 3 shows amplitude ratio: the highest-amplitude partial is assigned the value 1, and the other partials’ amplitudes are expressed relative to this (in multiphonic [3, 5, 8, 13], for example, the amplitude of the 8th partial is highest and the amplitude of the 5th partial is 91.2 per cent as high). The next columns show the partial number, the pitch (with cent deviations from equal temperament) and the frequency in Hz. Blank cells in the partials column mean that the frequency could not clearly be attributed to a partial (possibly noise from the recording, sympathetic vibration of a neighbouring string, etc.).
Referring back to Figure 2, a fundamental question arises: when using the theoretical model to predict pitch content, when should we stop implementing the steps of the algorithm – that is, how high up the harmonic series do the components influence multiphonic sound? Guettler says that for multiphonics on the double bass ‘the useful range of invocable harmonics seems to roll off around the twentieth’.Footnote 9 The existing fingering charts compiled in Figure 2 show that musicians seem to instinctively agree with this when notating multiphonic components. It is certainly possible to imagine physical reasons for this levelling off, such as string stiffness and string width relative to a partial's wavelength. My spectral analysis shows that partials in the 20s or even 30s can be fairly strong in relative amplitude and might thus have a colouristic role even if their specific pitch is not discerned as being part of the sound. The lower limits of contributing components, in particular the common exclusion of the open string and the 2nd harmonic, will be discussed below.
The question of upper limit can effectively be reformulated: how finely can cellists differentiate between the multiphonic branches shown in the second column of Figure 2? Take an example that would expand the Cello Map fingering charts: is it possible for a cellist to differentiate between two branches of the multiphonic [3, 5, 8, 13] to get [3, 5, 8, 13, 18] and [3, 5, 8, 13, 21] and is the difference audible? In terms of left-hand finger position, these multiphonics are theoretically approximately 1/2 cm apartFootnote 10 (or in cellistic terms, on the A-string between F4 + 30 cents and F4 + 53 cents) and are also strongly influenced by bowing conditions. It is hard to imagine cellists being able to distinguish between them; with some practice I managed to do this, but only in a very controlled context. On the monochord, where the string length is more than 3.7 times that of the cello,Footnote 11 the generous gaps between the harmonic nodes in question relative to the cello allow for more differentiation between multiphonic branches and [3, 5, 8, 13, 21] vs. [3, 5, 8, 13, 18] is easily differentiable. See Audio Example 4: Monochord [3, 5, 8, 13, 21] and [3, 5, 8, 13, 18].
In fact, the left-hand position of a multiphonic seems to be more diffuse: not so much a point as an area of feasibility. The areas of feasibility for [3, 5, 8, 13, 18] and [3, 5, 8, 13, 21] on the cello string probably overlap and the distinction between them might depend more on bowing factors than on left-hand position. Indeed, I will show below that the pitch content itself might also overlap. It seems that not only the cellist but the string itself has difficulty in distinguishing between these branches, and it would not be realistic to expand the Cello Map fingering charts in this case. In fact, it does not seem realistic to extend any of the eight multiphonics from the Cello Map fingering chart by further branching into higher harmonics. However, there are other extensions that can be made.
An important question related to branching is whether the string tends towards multiphonics with the fewest number of mutations or, in other words, those closest to the Fibonacci. A practical way of answering this question is to look at the results that musicians reported pre-theory. Almost all instrumentalists identified the Fibonacci multiphonic, so perhaps it is ‘easiest’ to produce. Technical factors may also play a role; for example, violinists, violists and cellists often describe a ‘tritone’ multiphonic (either [3, 4, 7, 11] or [3, 7, 10] situated about a tritone above the open stringFootnote 12) as easiest, the left-hand position being comfortable to locate and re-locate on the fingerboard surely influences this perception. This is one of the two multiphonics that Maurer suggests as feasible even for the violin and viola.Footnote 13 In the experiment with three cellists and three cellos, we played multiphonic IV[3, 7, 10] and tried to stabilize it so that we could hear all three main components. Of the nine recordings all shared the root [3, 7, 10]. Above this root six took a branch with the 13th harmonic and three took a branch with the 17th harmonic.Footnote 14 Although the 4th partial is generated by the algorithm, and has a high amplitude ratio in the analysis, I have excluded it from the notation because its octave relationship with the fundamental strongly reduces its audibility in the sound (instrumentalists also omit it from equivalent notations in Figure 2). This is proposed by Guettler, who suggests that all octave multiples of partials 2 and 3 will ‘hardly be heard’, even if they are strongly present in the spectrumFootnote 15 (there appear to be some exceptions to this proposal, however, as detailed below). The 17 highest-amplitude partials for three recordings of [3, 7, 10] are shown in Figure 4.
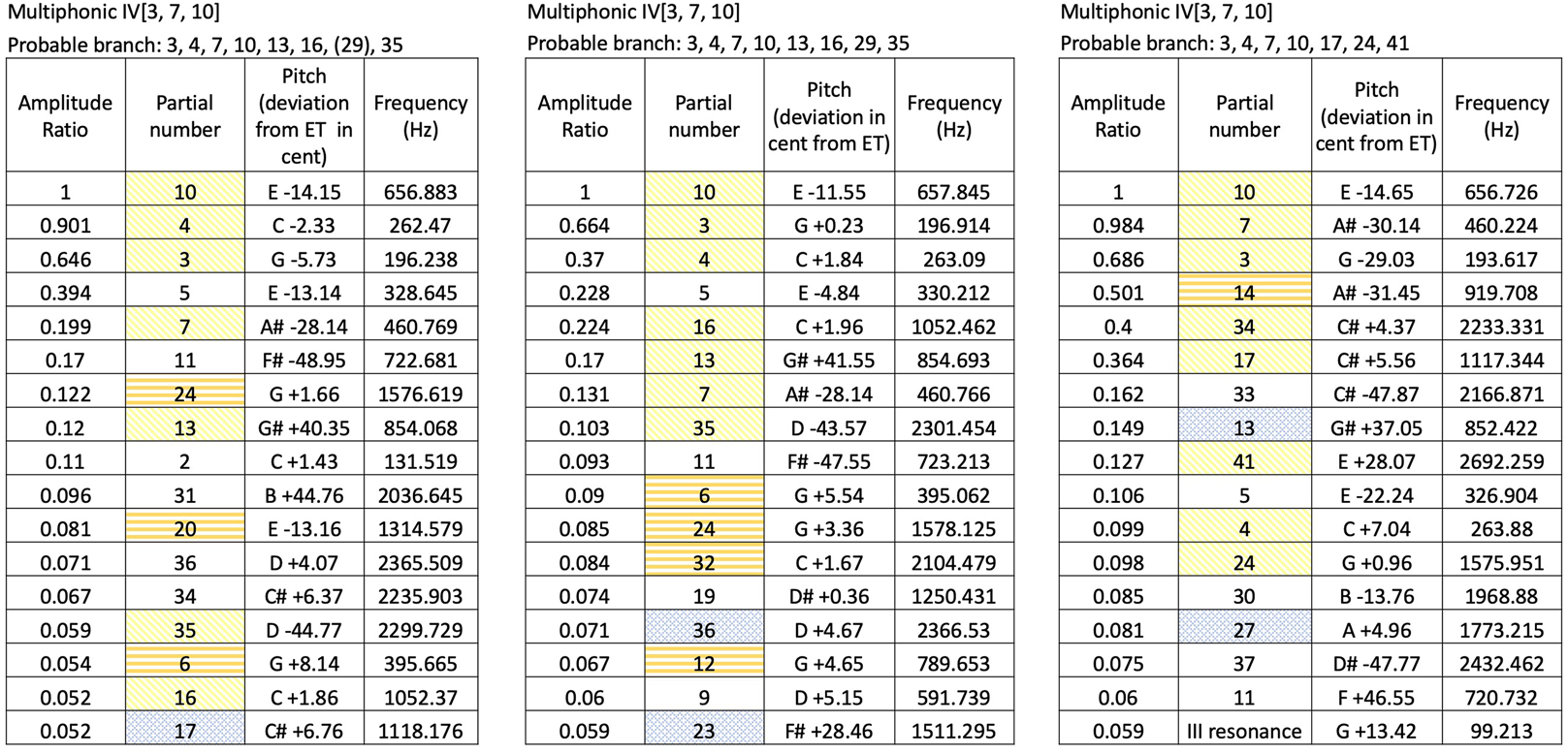
Figure 4: Three examples of IV[3, 7, 10]. See Audio Examples 5, 6 and 3.
Unless cellists are able to distinguish between branches with 13 and 17 (i.e. regions on the A string around E@4 + 37 cents and E@4 +3 cents) it seems advisable to remove 13 from my Cello Map notation [3, 7, 10, 13] because the 17th partial might be just as likely, or even more likely, to be present instead. In this case I decided that simplifying the notation was a realistic solution. In the three examples above, the first two appear to take the branch [3, 4, 7, 10, 13, 16, 29, 35] and the third [3, 4, 7, 10, 17, 24, 41]. In addition, there is evidence that harmonics of several different branches are simultaneously present (e.g. in Figure 4, the 17th partial in the first example, the 23rd and 36th partial in the second example, and the 13th and 27th partials in the third example, shown in dots in Figure 4). In fact, the categorization ‘other branches’ can be more simply defined as partials with nodal points close to the left-hand fingering position. This calls to mind Rita Torres's proposal that partials with nodal points within approximately 20 per cent of the wavelength from the touching position will to some extent be induced to soundFootnote 16 and Guettler's earlier characterisation of ‘filtering’ and ‘narrow clusters’ of partials. In any case it seems that, in addition to the theoretically predicted harmonics, certain partials are also present, and these are predictable, or at least unsurprising. This has an impact on choosing between realistic notations that are practical in a musical score or idealistic ones that try to give as much information about the sound as possible. Listening to the multiphonics in this context, it is possible both to hear that they share the main components and to discern differences in the upper partial band.
Similarly it seems logical to reduce the Cello Map notation [4, 5, 9, 13] to [4, 5, 9] since it is technically very difficult to distinguish between the branches 4, 5, 9, 13 and 4, 5, 9, 14. Of the nine recordings we made, five branched with the 13th and four with the 14th and in several recordings evidence of both branches were present. In contrast, on the monochord, this differentiation is easily controlled (see Audio Example 7). I think that the 4th harmonic should be included in the notation of this multiphonic because, contrary to Guettler's proposal, which seems appropriate in the previous example, I find it to be audible in the sound (see Audio Example 8). Its audibility in this multiphonic and not in [3, 7, 10] would be interesting to investigate more thoroughly and might have an impact on the issue of manipulating left-hand touching point to influence the relative amplitude of contributing harmonics.
Psychoacoustical elements surely also play a role in defining the upper limits of contributing harmonics: beating effects amongst the upper partials might limit their differentiability, critical bandwidth effects might make mid-range harmonics more discernible from one another, the louder lower components might mask the upper ones, etc. These aspects could be investigated in more detail.Footnote 17
In the listening test that I undertook with composition students at the Hochschule für Muisk in Basel, the students usually described the multiphonics as having 3 or 4 clear components, but they were able to ‘hear out’ 5 or 6 when prompted by interspersing the multiphonic recordings with recordings of individual harmonics. So this variable upper band of harmonics probably plays at least a colouristic role and, although it might not make practical sense to notate them, it may be musically interesting to describe a realm of possible pitches.
An additional pitch that might be present in a multiphonic is the vibrating string length between the left-hand ‘touching’ finger and the bridge. This seems to be particularly present on the cello's A-string (perhaps because of the relatively heavy left-hand finger weight relative to the lighter string) and can be clearly seen and heard in multiphonic I[5, 7, 12], shown in Figure 5. I recorded the 12th harmonic played at its 5th node for comparison. The touching-finger pitch is also very present in this sound. In addition to the 12th and 24th partials and the touching-finger pitch, partials 5 and 7 (the root components of multiphonic [5, 7, 12]) are also present (see Figure 5). It is interesting that, even when trying to isolate a harmonic, it contains a faint shadow of a multiphonic, and I will return to this later.
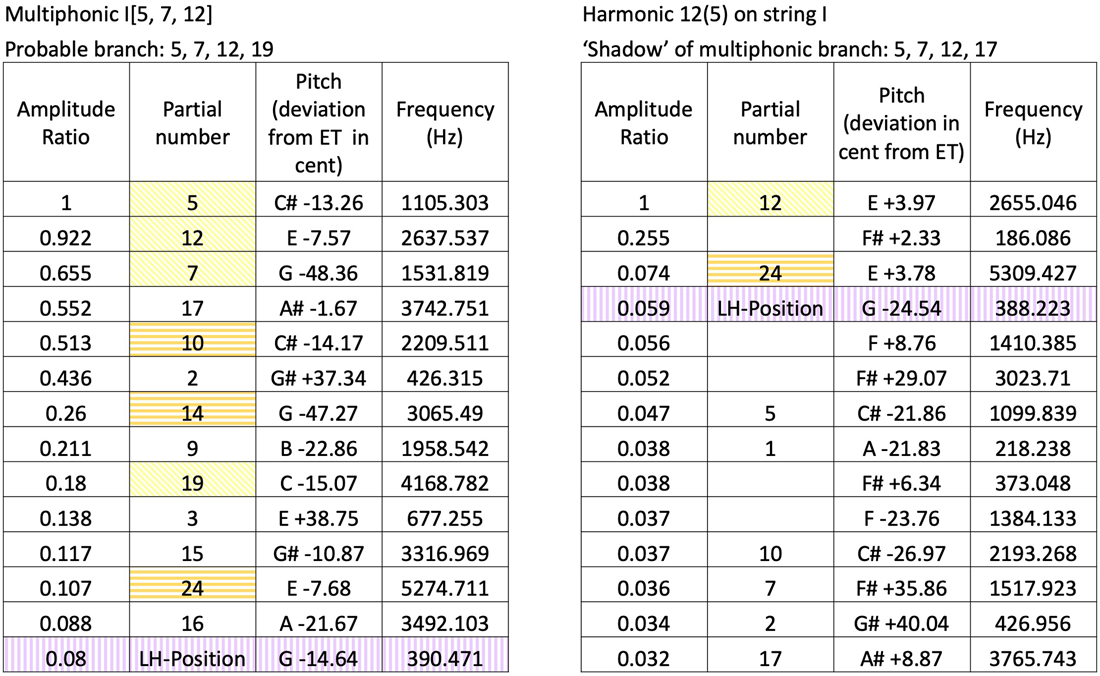
Figure 5: Multiphonic I[5,7, 12] and harmonic 12, touched at the 5th nodal point. Theoretically predicted multiphonic components/harmonic ‘fundamental’: 5,12, 7, 19. Octave multiples of these: 10, 14,24. Left-hand touching position: vertical stripes. See Audio Examples 2, 9.
In summary, there is certainly a moment at which the multiphonic branching of the string reaches a logical stopping point. This varies from instrument to instrument and might change in the future as instrumental technique develops. This has a significant impact on the notation recommended for a musical score, which requires simply the multiphonic ‘root’ within which there is no ambiguity for alternative branches. Nonetheless, the band of harmonics above this root influences sound quality. In addition to the concise notation required for a score, a more open representation of multiphonic pitch content that expands on the multiphonic ‘root’ to show likely additional sounds might be interesting for musicians. An example of such a notation is shown in Figure 6.
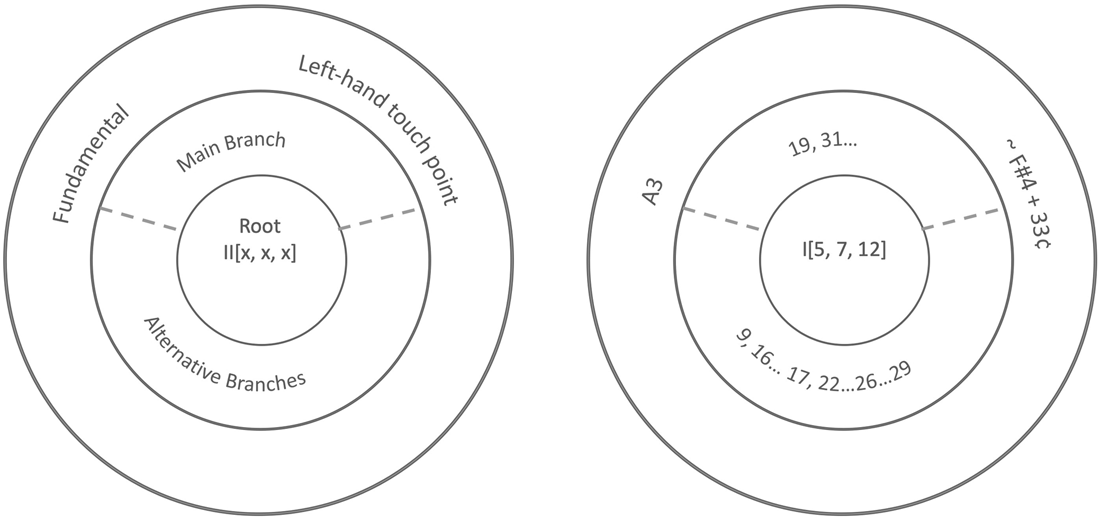
Figure 6: Left: the layout of a proposed visual representation of multiphonics. Right: an example for I[5, 7, 12].
Incremental Multiphonic Chains
In a special case of Walter's algorithm, mutating the series a maximum number of times eventually gives 1, 2, 3, 4, 5, 6, 7, 8, 9, 10 …. The series can get locked into what could be called a 1-chain since the increments are always 1. The chains theoretically always lead to the sum of the two last incremental entries: 1, 2, 3, 4, 5, 6, 7, 8, 9, 10, 19 … or 1, 2, 3, 4, 5, 6, 7, 8, 9, 10, 11, 12, 23 …. The chain could, theoretically, then continue in a Fibonacci manner; i.e. for the former, 10, 19, 29, 48, etc., or include further mutations. In practice, many of the lower entries in such chains are excluded from the sound, leaving approximately three incremental harmonics close to the stopping finger and the sum of the last two: in the above case 8, 9, 10, 19. The touching positions for the 1-chains tend asymptotically towards the end points of the string.
In reality the situation is slightly more complicated, as other additive partials appear to be present. This is similar to the situation described above: two multiphonic aggregates overlap or, simply, other partials with nodes in the vicinity are also activated. This is seen in Figure 7 for multiphonic II[9, 10, 11]. The sum of partials 10 and 11, 21, is fairly loud but 19 (9 + 10) is also present. Moreover, the relative amplitude of the 12th partial (which has a nodal point just above the touching finger) is also high.
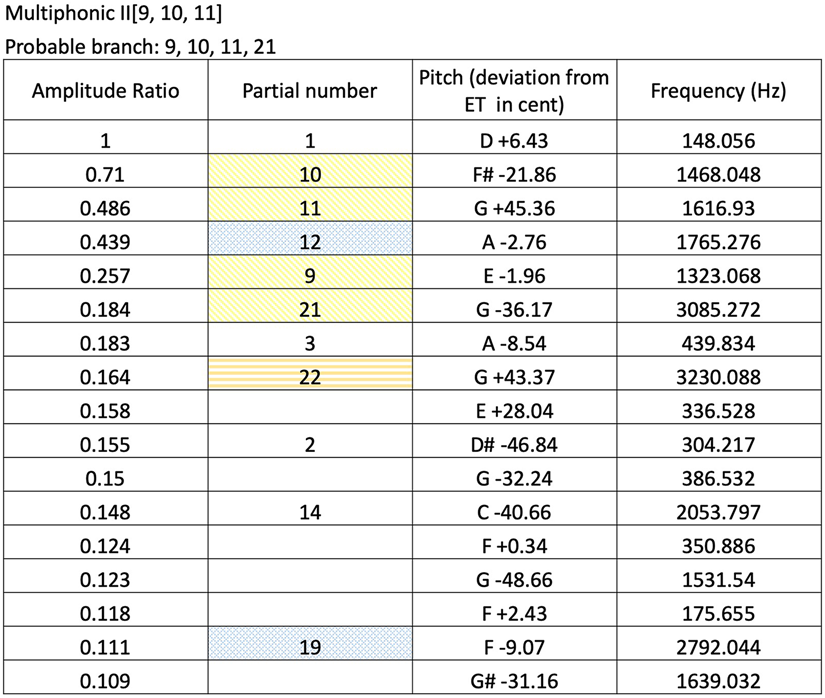
Figure 7: Multiphonic II[9, 10, 11]. With indications as above. See Audio Example 10.
There seems to be a technical advantage in playing relatively high 1-chain multiphonics, perhaps because the chain of the most audible harmonics have similar ideal bowing conditions (e.g. 7, 8 and 9). Fragile bowing conditions are an important aspect that make multiphonics difficult to play: it is necessary to find a bow stroke that constitutes a compromise between very different ideal bow speeds and pressures for the component harmonics (consider, for example, the contrasting ideal bowing conditions for harmonics 3, 5, 8 and 13). Consequently, multiphonics are usually restricted in dynamic range because the very bowing conditions that need to be altered to control dynamics are so narrow. In contrast, because of the main components’ similar ideal bowing conditions, the high 1-chains are a little more flexible in dynamics and, in particular, loud dynamics and effects such as sforzando are possible. The cello and double bass have the advantage of being able to ‘force’ the 1-chains to sound by bowing between the left-hand finger and nut; the ‘recipe’ for bowing conditions is simply something that accommodates n, n + 1, n + 2 but has enough bow force and a slow enough bow to allow the fundamental through; these conditions seem easier to meet when bowing ‘behind’ the touching finger.
When do we start describing multiphonics as 1-chains? Even the Fibonacci multiphonic starts 1, 2, 3 and simple mutations contain short + 1 incremental series such as 1, 2, 3, 4, 7. It could be defined as the moment when the band of three or more incremental harmonics in the form n, n + 1, n + 2 is clearly the strongest part of the sound. For the cello this coincides with the moment when it is possible to comfortably bow on the nut-side of the stopping finger (around [7, 8, 9]). This is a porous definition that changes from instrument to instrument, and will be interpreted differently, but it is nonetheless loosely applicable. Guettler and Thelin categorise multiphonics that are produced by bowing on the nut-side of the finger differently to those that are produced on the bridge-side because the force spectrum at the bridge is more similar to conventional bowing for the former than the latter. For this reason, Guettler explains, the fundamental (open string) is usually emphasised in the sound.Footnote 18 This can clearly be heard in the recordings and is also seen in the analysis above, where the open string is the loudest component.
1-chains bowed behind the stopping finger have a characteristic noisy quality. This could be connected to the prominence of the open string, the intonation of which is very variable (discussed further below), or it could be noise generated by small variations in the vibration period caused by overpressure of the slightly forced bow stroke.Footnote 19 Musicians often identify a ‘fluttering’ of the higher harmonics in 1-chains. This could be a beating between the partials in this close upper band and/or connected to the technique of bowing behind the finger. This oscillatory effect is described by Marc Dresser regarding double bass multiphonics.Footnote 20 In an early reference to multiphonics that pre-dates his more detailed analysis, Guettler also describes them as ‘swift changes between the fundamental and the harmonic sound’.Footnote 21
Because the additive partials are usually very high, I recommend notating the multiphonic root of 1-chains as the three dominant components, e.g. [9, 10, 11]. The 1-chains offer a wide extension to the Cello Map fingering charts and are viable even for high harmonic groupings such as [18, 19, 20].
It is also possible to generate 2-chain multiphonics of the form 1, 2, 3, 5, 7, 9, 11, 13 …. The left-hand fingering for 2-chains tends asymptotically towards the mid-point of the string. These are harder to control with the bow than the 1-chains (bowing behind the finger is not an option at the mid-point of the string). Josel identified a much-mutated 2-chain in his fingering charts for guitar [2, 11, 13, 15, 17].Footnote 22 In 2-chains the second harmonic is prominent, which is unusual in multiphonics. Since the left-hand finger is close to the string's mid-point, odd-numbered partials of the fundamental seem to be filtered out and the second harmonic is strongly audible. Similarly there exist other types of chains (e.g. 3-chains 1, 2, 3, 5, 8, 11, 14, 17, 20, 23, … tending towards 1/3 and 2/3 of the string length and 5 chains 1, 2, 3, 5, 8, 13, 18, 23, 28, 33, … tending towards 2/5 and 3/5 of the string length, and within any multiphonic there can exist blocks of three or four incremental partials with the same additive component). Although these appear to be special cases with many mutations the similar ideal playing conditions within narrower bands of harmonics, and the capping of realistic contributing partials for the bowed cello string at around the 20th, mean that it is not uncommon to see evidence of such chains.
Balance: Relative loudness of components
The relative amplitude of the partials in the multiphonics I recorded varied considerably: in some cases the second-loudest partial was less than half the amplitude of the first and in other cases it was almost the same. Since all the samples had a multiphonic-like sound, this indicates that the balance between components does not play a significant role in identifying multiphonics as such. In Figure 8, several multiphonics with different amplitude ratio profiles are shown. The amplitude of the second loudest partial relative to the first varies from 47.2 per cent to 92.5 per cent. The amplitude of the 10th loudest partial relative to the first varies from 9.6 per cent to 39.6 per cent. The rate of reduction in amplitude ratio also varies.
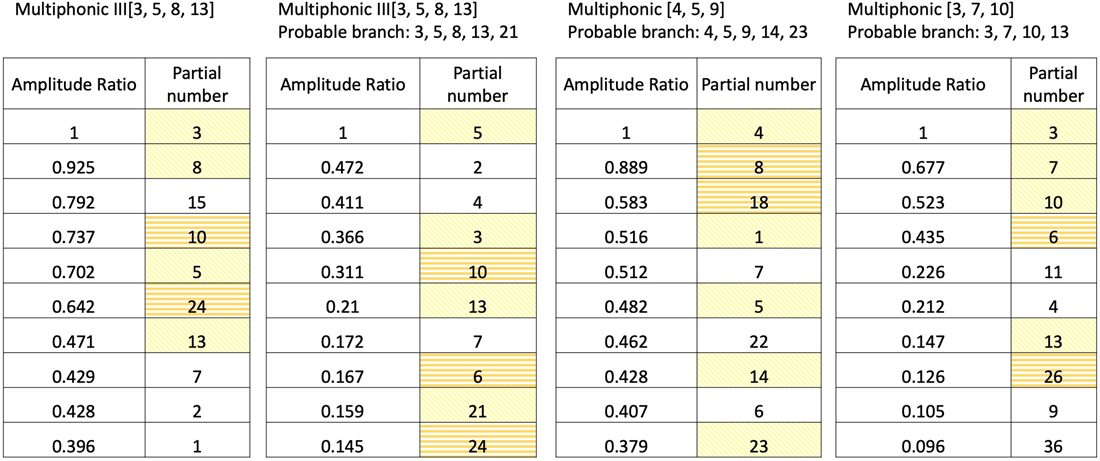
Figure 8: The 10 highest-amplitude partials of four multiphonics and their relative amplitudes (indications as above). See Audio Examples 11, 12, 13, 14.
It is important to note that the measurement of partials’ relative amplitude can be deceptive: if harmonic multiples of partials are also present (as is usually the case), the perceived loudness of the harmonic of which the former partial is the fundamental will be enhanced. For example, if partials 6 and 3 both have relatively high amplitudes, then the 3rd harmonic will be perceived as louder than the amplitude of the 3rd partial. Similarly the ‘missing fundamental’ effect suggests that the fundamental can be perceived even if it is barely present, as explained by Benade: ‘we get a strong sense of pitch from a tone made up of a set of harmonic partials, even if several of these (including the fundamental component) are missing’.Footnote 23 This is certainly true in multiphonics where the fundamental is often very low in terms of relative amplitude but usually perceived to be present (although, as mentioned above, not necessarily perceived as ‘important’).
Although, in terms of categorising a sound as a multiphonic or not, the relative amplitude does not seem to be an important metric, it strongly influences timbre. All of the Basel listening test participants agreed with the statement ‘One or two pitches are significantly louder and dominate the sound’ and no-one described multiphonic components as ‘well-balanced in terms of loudness’. The results with three cellists/cellos showed a large range in relative amplitude drop off and, in addition, the dominating component varied. Balance can be controlled to an extent with the left-hand position and the bow by manipulating the playing conditions to favour one component (a particularly fast bow stroke to emphasise the higher partials, moving the left hand towards a specific node to increase the associated harmonic's relative amplitude, etc.). As discussed above, since the bowing and fingering conditions for multiphonics are restricted, these changes have to be made within a narrow range.
Outside this range, the multiphonic breaks up into one of its harmonic components or shifts to a neighbouring multiphonic. Tilting the bow (or using a thinner, denser plectrum) also seems to favour higher partials relative to the lower components. Playing sul ponticello increases the relative amplitude of the high partials and their octave multiples. This is only the case for bowing positions fairly close to the bridge since the factor ‘point of contact’ for multiphonics is far more complicated than under usual bowing conditions. This is easily proved: consider the open string with the region of maximal sul tasto at the mid-point and maximal sul ponticello at the bridge and nut. The 2nd harmonic has two maximal sul tasto regions at 1/4 and 3/4 of the string length, and sul ponticello regions at the string ends and its mid-point. The 3rd harmonic has three maximal sul tasto regions at 1/6, 1/2 and 5/6 and sul ponticello regions at 1/3 and 2/3 and the string ends, etc. It is easy to see how a superimposition of several harmonics within a multiphonic equates to a complex pattern in terms of sul ponticello and sul tasto. It is also easy to imagine unintentionally enhancing or filtering out some components with the choice of right-hand contact point. Moreover, it is difficult to imagine intentionally using contact point to control colour reliably. In Figure 9 it can be seen that a relatively extreme sul ponticello position is the only position that has an easily predictable effect on overtone content. This was also confirmed in my analysis, in which the octave multiples of predicted partials dominated.
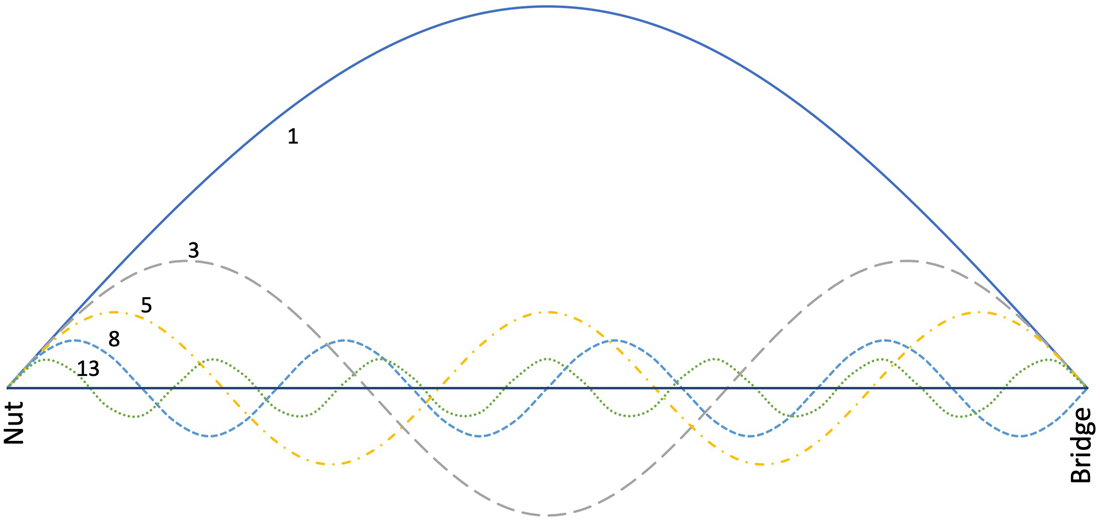
Figure 9: The wave forms of the open string, 3rd, 5th, 8th and 13th partials. The only common sul ponticello regions are at the nut and bridge.
Arash Yazdani has undertaken some comparative analysis for piano multiphonics on influencing overtone content by changing playing conditions at the left-hand touching finger. He compared four situations: touching with the tip of the index finger, the fingernail, pinching the string between thumb and index finger and using two touching fingers. He found that the former two techniques induce more overtones and the latter two a darker sound with fewer but more distinct peaks.Footnote 24 It might be possible to adapt these techniques to cello multiphonics.
Conversely, it is sometimes very difficult to produce ‘pure’ harmonics that are completely free from multiphonic components. On the cello this is particularly true for nodal points of mid-high harmonics in the middle region of the string (in particular, not the 1st or n-1th nodal point) and is emphasised for plucked multiphonics.Footnote 25 A faint shadow of multiphonic components was, for example, seen in the recording of the 12th harmonic at the 5th node above (see Figure 5 above). In fact, it might be considered that there is a point on each string instrument (dependent upon the playing conditions) where it is virtually impossible to play a high harmonic in isolation without some neighbouring harmonics sounding in a ‘shadowy’ multiphonic. This is exemplified in Olsson, Svensson and Bauck's piano harmonics resource where, on the lower strings, the harmonics have a strong multiphonic-like character. The authors give the example of the 11th harmonic at nodes 2/11 and 3/11 being ‘subtly coloured by adjacent harmonics (17th and 16th, and 15th and 18th, respectively)’ but consider this technique as separate from intentionally producing multiphonics.Footnote 26
Another important aspect of balance is perceived in pizzicato. It is a clearly audible characteristic of plucked multiphonics that relative loudness changes during the decay. This was mentioned by Josel and Tsao.Footnote 27 This effect is particularly clear on the monochord and sounds like a slow beating back and forth (see Audio Example 15). The fast decay of plucked cello multiphonics make this effect more difficult to hear but it is nonetheless perceivable in the following recording (see Audio Example 16). It would be very interesting to understand more about the effects on the strings or within instrument bodies that create this apparent input of energy into the system during the decay.
Intonation
Analysing the intonation of multiphonics generates results that are not necessarily directly audible but explain some characteristic aspects of multiphonic sound. To summarise: on a cello string upper partials should become progressively sharper relative to the harmonic seriesFootnote 28 owing to a string's bending stiffness. Guettler found the intonation of double bass harmonics to be ‘mathematically correct or sharp’.Footnote 29 Daniel Russel reported inharmonicity in fairly long, thin guitar strings to be negligible.Footnote 30 A brief analysis that I made of cello harmonics shows that the partials most relevant to multiphonics are almost exactly in tune. For example, I recorded the open G string and the 13th harmonic on the G string. Over two bow strokes, the fundamental pitch of the open string wavered from around -6 cents to around -3 cents. For the 13th harmonic, despite the weight of the left-hand finger touching the string and the potential interference that might be expected to cause, the 1st, 2nd 3rd and 4th partials (relative to the open string, the 13th, 26th, 39th and 52nd) were never more than a few cents away from harmonic tuning. The sharpening effect caused by string stiffness might, therefore, be expected to be insignificant in the range of harmonics that dominate multiphonics.
While this seems to be true for cello multiphonics an analysis of intonation presents a surprising picture. The intonation of the open string is extremely unstable, fluctuating strongly between sharp and flat within a few seconds. Moreover, the 2nd partial is often also volatile and can be very out-of-sync with the fundamental (sharp while the latter is flat and vice versa). The intonation of the higher partials is more stable and seems to improve progressively for ascending partials. Mid and high-range partials are in tune according to the harmonic series. Some comparative results can be seen in Figure 10a and b.
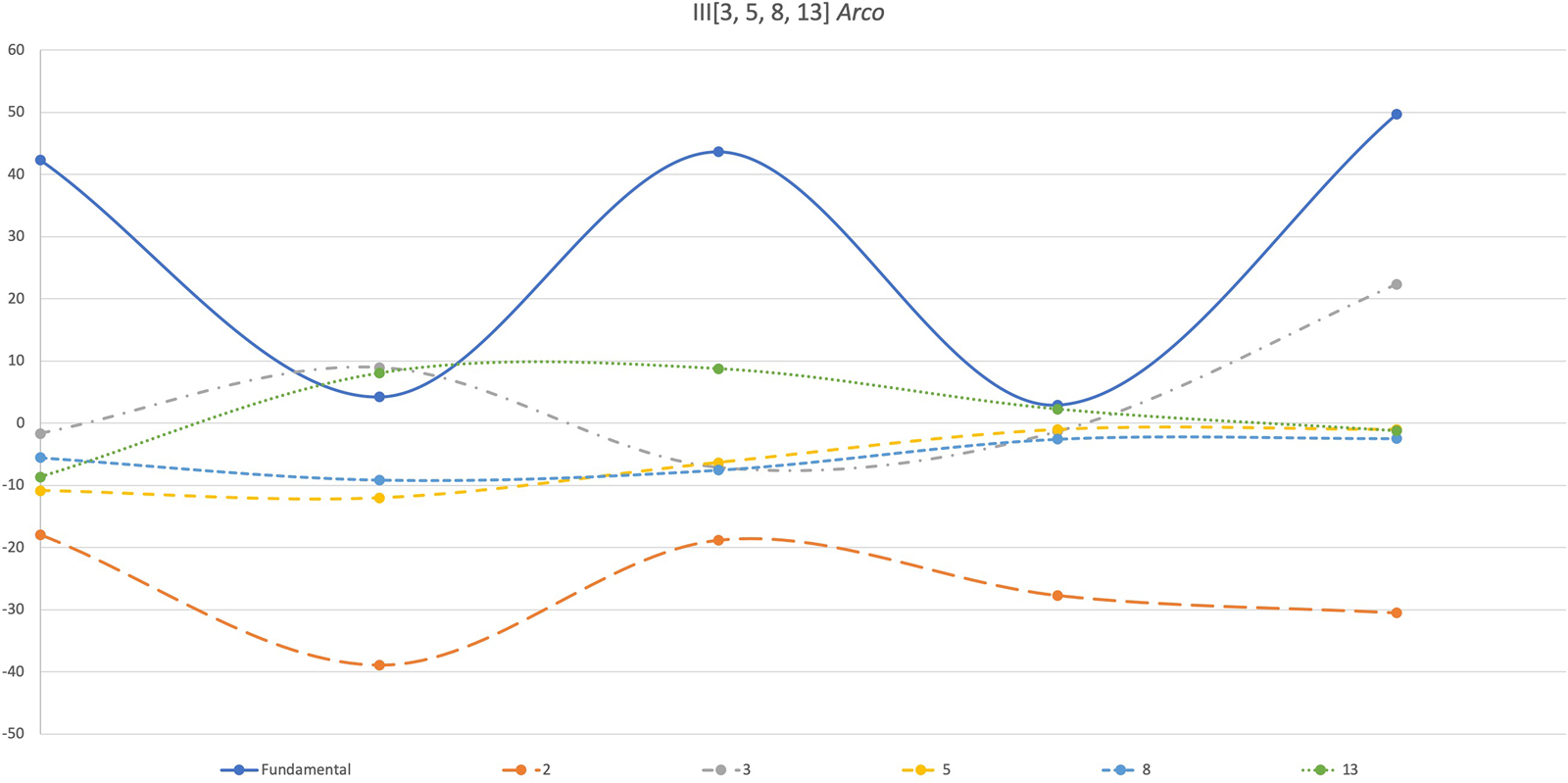
Figure 10a: The intonation of the fundamental, 2nd partial and components of III[3, 5, 8, 13], played arco, measured at regular intervals throughout a bow stroke. The intonation of the fundamental and 2nd partial vary greatly but are fairly synchronized with one another. The other partials have much more stable intonation. See Audio Example 12. Key: unbroken line = fundamental; thick dash = 2nd partial; dash dot = 3rd partial; medium dash = 5th partial; thin dash = 8th partial; dots = 13th partial.
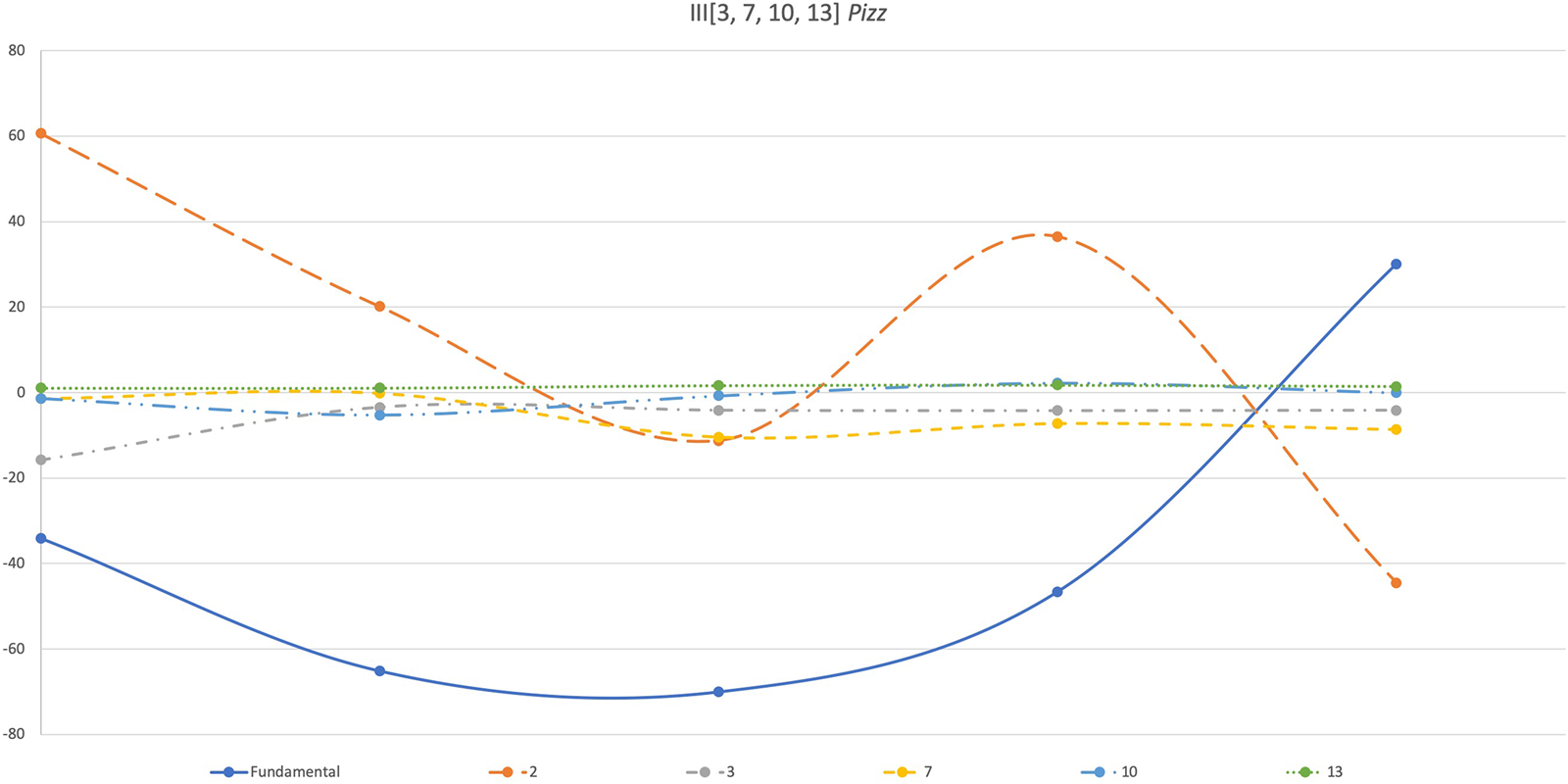
Figure 10b: The intonation of the fundamental, 2nd partial and components of III[3, 7, 10, 13] played pizzicato measured at regular intervals throughout the decay. As above, the intonation of the fundamental and 2nd partial vary greatly, and the intonation of the other partials, particularly the 13th is much more stable. Key: unbroken line = fundamental; thick dash = 2nd partial; dash dot = 3rd partial; thin dash = 7th partial; dash dot dot = 10th partial; dots = 13th partial. See Audio Example 17.
Effects that operate in a bowed string to sharpen or flatten the pitch under high bow pressure/velocity have been investigated in the context of subharmonics and pitch flattening (e.g. the delayed triggering of the string's release from the bow causing anomalous low frequencies,Footnote 31 the increased tension of a stretched string under high pressure causing sharpening effects,Footnote 32 and the ‘corrective’ effect of the bow on intonation by ‘resharpening’ the Helmholtz corners of the stringFootnote 33). Some of these effects could be at play here but, because pizzicato multiphonics on the cello (and plucked guitar and harp multiphonics) show similar results, other fundamental aspects of vibration must also be influential. It would be interesting to try to unravel these.
In addition, for 1-chain multiphonics bowed between stopping finger and nut, it seems that the fundamental is more in-tune when played directly behind the left-hand finger, and less in-tune when bowed a short distance away from the stopping finger. It might be possible to influence intonation here.
For me the most interesting aspect of the fluctuations in tuning of the fundamental and 2nd harmonic is that they are not heard as such. In the listening test with composition students at the Hochschule für Musik Basel, all of the students described the pizzicato multiphonic and most described the other multiphonics as having stable intonation. However, the listeners were much more divided as to whether they could describe the multiphonics as ‘chords’ or ‘complex sounds’ and whether they would describe them as ‘consonant’ or ‘dissonant’. These questions split the participants fairly evenly. It could be that the fluctuations in intonation function to destabilize the perceived sound and play a more important role in characterising the colour of multiphonics than defining its pitch. This could be connected to the description of a bell-like quality that so often arises when discussing multiphonics with musicians (although the overtones of bells are not harmonically related). Perhaps the detuning of the lower partials, which are a little too far apart to beat strongly with one another, helps the ear to sieve them out of a multiphonic and even plays a role in enabling multiphonics to be heard as chord-like sounds. Or perhaps this is a reason why the fundamental is often not regarded as being part of the multiphonic but rather a separate, background entity.Footnote 34
The detuning of the fundamental and 2nd harmonic might also create the sense of ‘harmonic dissonance’ that Sethares describes, extrapolating from James Tenney, and may contribute to this perception of a bell-like or ‘dirty’ sound.Footnote 35 It is the case that, while it is hard to define a clear ‘recipe’ for changing the contact point of the bow on the string, bowing close to the bridge produces distinctly cleaner-sounding multiphonics than bowing sul tasto. It could be that playing in the sul tasto region emphasises the open string sound and enhances the feeling of dissonance by increasing the relative amplitude of the detuned and unstable fundamental. I was disappointed that neither my analysis not background reading could offer a proposal to explain an aspect of sound that I find to be so distinctive and characteristic of multiphonics. Again, this would be an interesting area to explore further. This has technical implications because bowing further from the bridge makes multiphonics easier to produce, as there is less perceived stiffness in the string compared to areas close to the bridge.Footnote 36 In this case the unintended or intended consequence of the destabilisation of the sound should be taken into account: the destabilised sound might, of course, be a ‘compromise’ worth making if the multiphonic is more likely to speak.
Many of the aspects of multiphonics that I have presented here are inconclusive and pose questions that I consider worth exploring in the future. The increased popularity of the technique is already leading to technical extensions. Artificial multiphonics enabling glissando effects, transitions between multiphonics and harmonics, and subtle changes in balance that emphasise certain components continue to be explored to musical effect. Some technical aspects will remain restrictive, however, because of the narrow range of feasible playing conditions: for example, double stops (especially for multiphonics with left-hand fingering positions that do not lie parallel on two strings), forte and sforzando (with the exception of 1-chain multiphonics bowed between finger and nut), preparations (especially those applied directly to the string) and staccato or multiphonics played in quick succession (because it takes a little time to establish a bowed multiphonic). Similarly, in an ensemble context, it is very easy for the components of multiphonics to be masked by other sounds. Thus, we rely on the technical development and the musical imaginations of both composers and performers for cello multiphonics to become musically rich and technically standardized.
The publication of the upcoming smartphone application and repository, including updated fingering charts, will be announced on www.cellomap.com.
Appendix
Audio Examples (all at https://soundcloud.com/musikforschungbasel/sets/audio-examples-tempo-string-multiphonics-ellen-fallowfield)
Audio Example 1 III[3, 5, 8, 13] KK
Audio Example 2 I[5, 7, 12] EE
Audio Example 3 IV[3, 7, 10] MM
Audio Example 4 Monochord [3, 5, 8, 13, 21] and [3, 5, 8, 13, 18]
Audio Example 5 IV[3, 7, 10] EE
Audio Example 6 IV[3, 7, 10] KK
Audio Example 7 Monochord [4, 5, 9, 13] and [4, 5, 9, 14]
Audio Example 8 III[4, 5, 9] KE
Audio Example 9 I 12(5) EE (the 12th harmonic on the A string, played at the 5th node)
Audio Example 10 II[9, 10, 11] EE
Audio Example 11 III[3, 5, 8, 13] EK
Audio Example 12 III[3, 5, 8, 13] EM
Audio Example 13 III[4, 5, 9] EE
Audio Example 14 IV[3, 7, 10] KM
Audio Example 15 Monochord [5, 6, 11, 17] and [5, 6, 11, 16]
Audio Example 16 III[3, 7, 10] pizz EE
Audio Example 17 III[3, 7, 10] pizz EE
Multiphonic notation as described above.
The letters E, K and M describe which cellists (Ellen Fallowfield (E), Karolina Öhman (K) and Mathilde Rémy (M)) are playing on whose cello. For example, EK = Ellen Fallowfield playing on Karolina Öhman's cello, etc.
Conditions of recordings
Recording Session 1 (Audio Examples 9, 16, 17)
Cello: Ellen Fallowfield, recording: Omri Abram, microphones: Stereo omni pair, 2 DPA 4006; MS pair, Schoeps MK5 and MK 8.
Recording Session 2 (Audio Examples 1–3, 5, 6, 8, 10–14)
Cello: Ellen Fallowfield, Karolina Öhman, Mathilde Rémy, recording: Paul Clift, microphones: 2 Neumann KM184.
Recording Session 3 (Audio Examples 4, 7, 15)
Monochord: Ellen Fallowfield, recording: Francesca Verga, microphones: Zoom H4n recorder.
Monochord: manufacturer: unknown, date of manufacture: 1971, dimensions of wooden body: 22.5 × 27.5 × 260.7 cm, string length 240 cm, pitch of vibrating string: approx. 60.8 Hz (B1 −26 cents). We used one of 5 strings. Due to the sensitivity of the structure, we decided not to try to tune the strings and simply measured the intonation of the string that we had chosen to play.
My thanks go to Keitaro Takahashi for building the analysis programme; Caspar Johannes Walter and Erik Oña for their scientific advice; Paul Clift, Omri Abram and Francesca Verga for making the recordings; cellists Karolina Öhman and Mathilde Rémy; Michel Roth and his composition class at the Hochschule für Musik Basel for undertaking the listening test; David Eggert for lending his monochord and the Hochschule für Musik Basel and Swiss National Science Foundation (BRIDGE Proof of Concept) for the financial support.