Book contents
- Clinical Mitochondrial Medicine
- Clinical Mitochondrial Medicine
- Copyright page
- Contents
- Contributors
- Preface
- Abbreviations
- Section 1 Introduction to Mitochondrial Medicine
- Chapter 1 Mitochondria in Health and Disease
- Chapter 2 Clinical Approach in Children
- Chapter 3 Clinical Approach in Adults
- Chapter 4 Laboratory Investigation of Mitochondrial Diseases
- Chapter 5 Clinical Genetics of Mitochondrial Diseases
- Chapter 6 Clinical Management of Mitochondrial Diseases
- Section 2 Systems Involved in Mitochondrial Diseases
- Index
- Plate Section (PDF Only)
- References
Chapter 4 - Laboratory Investigation of Mitochondrial Diseases
from Section 1 - Introduction to Mitochondrial Medicine
Published online by Cambridge University Press: 28 April 2018
- Clinical Mitochondrial Medicine
- Clinical Mitochondrial Medicine
- Copyright page
- Contents
- Contributors
- Preface
- Abbreviations
- Section 1 Introduction to Mitochondrial Medicine
- Chapter 1 Mitochondria in Health and Disease
- Chapter 2 Clinical Approach in Children
- Chapter 3 Clinical Approach in Adults
- Chapter 4 Laboratory Investigation of Mitochondrial Diseases
- Chapter 5 Clinical Genetics of Mitochondrial Diseases
- Chapter 6 Clinical Management of Mitochondrial Diseases
- Section 2 Systems Involved in Mitochondrial Diseases
- Index
- Plate Section (PDF Only)
- References
Summary
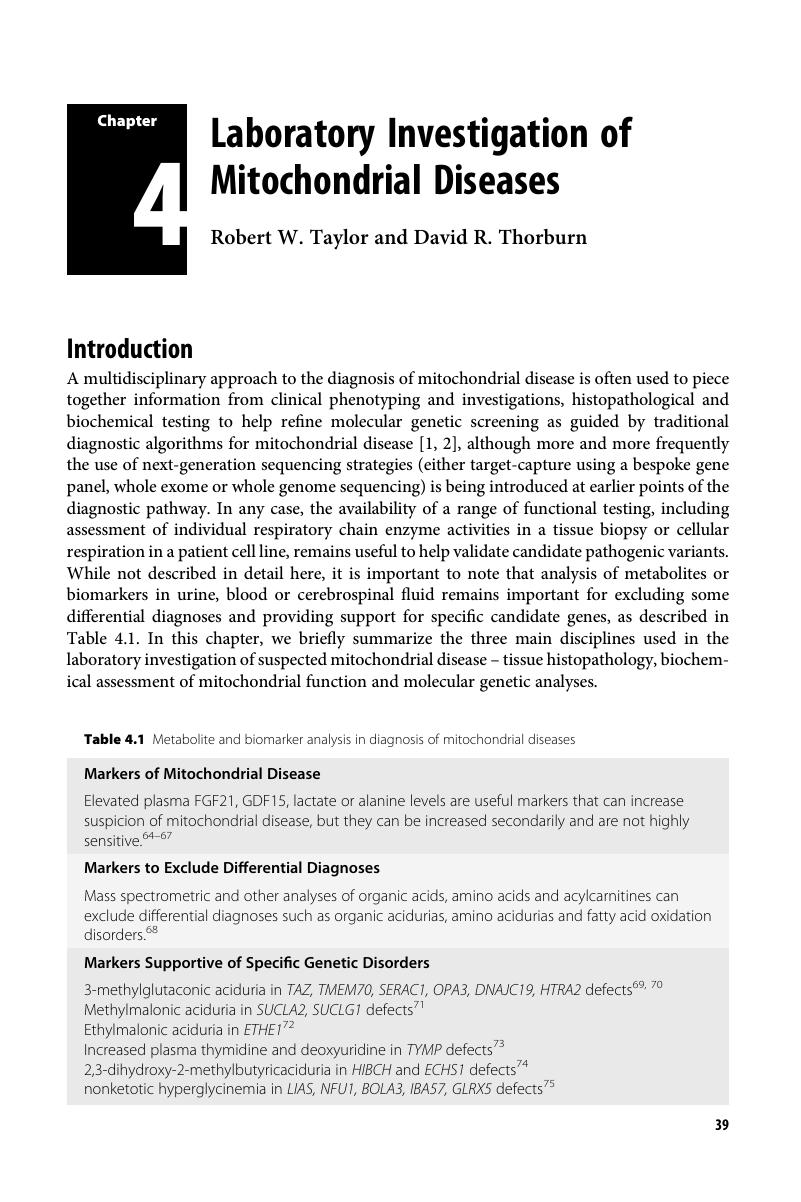
- Type
- Chapter
- Information
- Clinical Mitochondrial Medicine , pp. 39 - 51Publisher: Cambridge University PressPrint publication year: 2018