II - Theory, instrumentation, and laboratory studies
Published online by Cambridge University Press: 05 May 2015
Summary
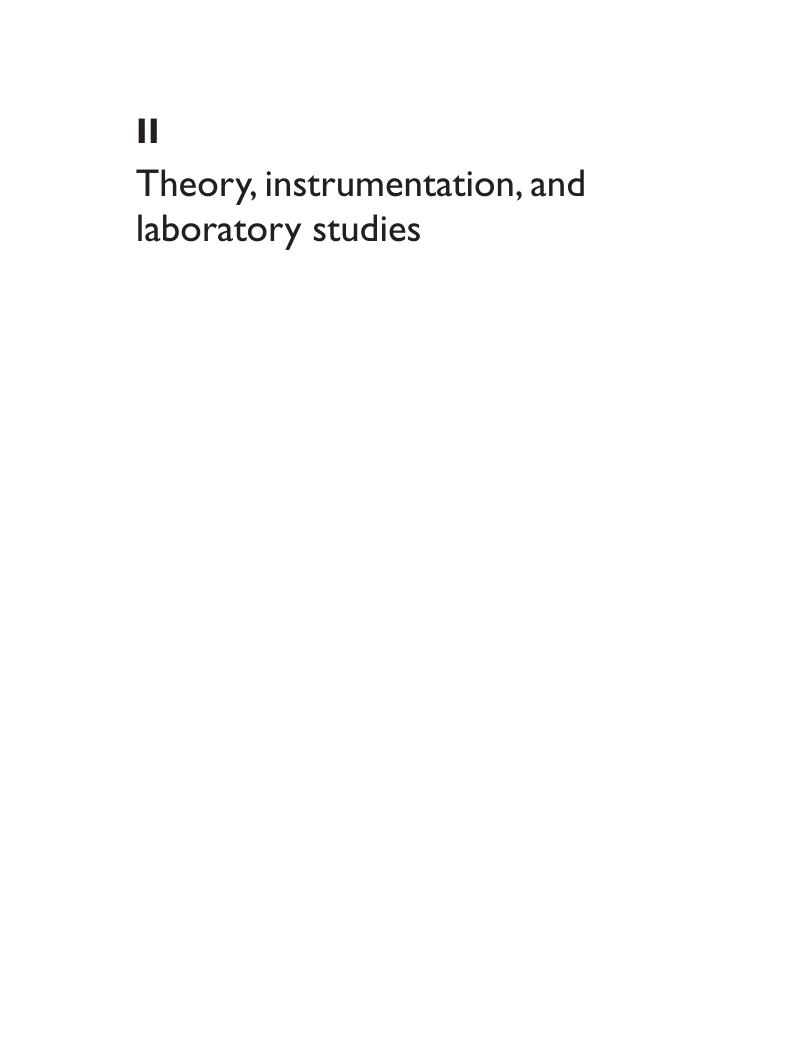
- Type
- Chapter
- Information
- Polarimetry of Stars and Planetary Systems , pp. 11 - 144Publisher: Cambridge University PressPrint publication year: 2015