1. Introduction
Clay minerals are used in different applications owing to their high cation exchange capacity and high surface area. Bentonite clay consists mainly of crystalline clay minerals belonging to the smectite group, which are hydrous aluminum silicates containing Fe and Mg as well as either Na or Ca. Bentonite forms from the alteration of volcanic glass to clay minerals which requires hydration (combination or taking up of water) and a loss of alkalis, bases, and possibly silica, with the preservation of the textures of the original volcanic glass. Bentonite is mainly composed of montmorillonite (Uddin, Reference Uddin2008) and other minor nonclay minerals, in which it has an overall negative charge either in tetrahedral sheet or octahedral sheet form. Montmorillonite, a smectite clay mineral composed of three layers, has a 2:1 ratio of Si4+ tetrahedral to Al3+ octahedral sheets. The interlayer, formed by one octahedral sheet sandwiched between two silica tetrahedral sheets, between its units is composed of positive cations and water molecules making them excellent supporting materials for nanoparticles (Motshekga et al., Reference Motshekga, Ray, Onyango and Momba2013).
The structure of smectite minerals allows multiple positional or elemental substitutions within its lattice. Such substitutions induce a deficiency in net positive charge, which is thereby balanced by exchangeable cations (Olegario & Felizco, Reference Olegario and Felizco2017). The members of the smectite minerals are classified based on the species and location of the cations in the crystal structure. When trivalent cations occupy two-thirds of the octahedral sites, these minerals are called dioctahedral smectites which include montmorillonite, beidellite, and nontronite. When divalent cations occupy all three octahedral sites, these are called trioctahedral smectites. Examples of which are saponite, sauconite, and hectorite (Odom, Reference Odom1984).
2. Objectives
This work is an extensive characterization of Philippine natural bentonite using various characterization techniques which include X-ray diffractometer (XRD), chemical analysis, scanning electron microscope (SEM), thermogravimetric-differential scanning calorimetry (TG-DSC), Fourier transform infrared (FTIR), and cation exchange capacity (CEC) measurements. Although there are already several studies on Philippine natural bentonite, most of which are centered on the applications of bentonite. Dahonog et al. (Reference Dahonog, Tugado, Olegario-Sanchez and Vasquez2019) studied the antibacterial activity of plasma-treated Cu-bentonite nanocomposites. Olegario and Felizco (Reference Olegario and Felizco2017) investigated the structural properties of amorphous Philippine bentonite clay and its potential use for topical applications. In this study, the CEC was measured, and the pH was determined. Microbial analysis was done alongside the XRD characterization of the mineral. Taaca et al. (Reference Taaca, Dahonog, Argayosa, Rubio and Olegario2019) assessed the antimicrobial and cytotoxic activities of ion-exchanged Ag-modified bentonite. Cruz and Diaz (Reference Cruz and Diaz2019) studied the adsorption of Cu(II) onto Fe(III)-modified montmorillonite. As stated, most studies revolved around the applications of Philippine bentonite. Therefore, there is a need to make a separate study on the characterization of the material. This work is much more comprehensive in terms of characterization techniques than the rest of the existing papers.
3. Methodology
Bentonite powder samples were provided by LITHOS Manufacturing, OPC. The bentonite was mined from Mangatarem, Pangasinan in Luzon, Philippines. The powder is finely ground with sizes ranging from 100 to 200 mesh. It has a brown to reddish color and has a true specific gravity of <2.2. The structure and crystallinity of the raw bentonite sample were analyzed using an XRD (Shimadzu XRD-7000) using Cu-Kα radiation from 2° to 70° 2θ. The morphology of the bentonite clay was examined using an SEM (Shimadzu SSX-550) with an acceleration voltage of 15.0 kV. Chemical tests were separately done to confirm the amount of each chemical component present in the Philippine bentonite samples. The thermal behavior and stability of the raw bentonite were investigated using DSC (TA Instruments DSC Q10) at a heating rate of 10.00°C/min from room temperature up to 1,000°C. The vibration bands present in the samples’ transmittance spectrum were analyzed using an attenuated total reflectance FTIR (ATR-FTIR) analyzer (Perkin Elmer FTIR Spectrometer) from 4,000 to 650 cm−1. Cation exchange capacity measurements were conducted using ammonium acetate and barium acetate methods.
4. Results and Discussion
4.1. X-ray diffractometry
Figure 1 shows the X-ray diffractogram of the raw bentonite sample used in this study. It can be observed that the sample is mainly composed of hectorite (amcsd 0015819) and montmorillonite (amcsd 0002868) from the smectite group and mordenite (amcsd 0003444) from the zeolite minerals. However, the amount of each component cannot be discerned as the peaks of each component overlap each other. Small amounts of nontronite (amcsd 0007180), and other minerals such as kaolinite (amcsd 0017947), feldspar (amcsd 0000436), and mica (amcsd 0000836) were also present in the sample. Calcite, cristobalite, and quartz, which are possibly carcinogenic (Modabberi et al., Reference Modabberi, Namayandeh, López-Galindo, Viseras, Setti and Ranjbaran2015) were not detected in the sample. The results agree well with the XRD patterns of the Philippine bentonite in previous studies (Olegario & Felizco, Reference Olegario and Felizco2017; Taaca et al., Reference Taaca, Dahonog, Argayosa, Rubio and Olegario2019). The bentonite sample used in the study share several common components with the Philippine natural zeolite reported in our previous studies as they are mined from the same area in Mangatarem, Pangasinan (Gili & Olegario, Reference Gili and Olegario2020; Gili, Olegario-Sanchez, & Conato, Reference Gili, Olegario-Sanchez and Conato2019; Gili, Pares, et al., Reference Gili, Pares, Nery, Guillermo, Marquez and Olegario2019).
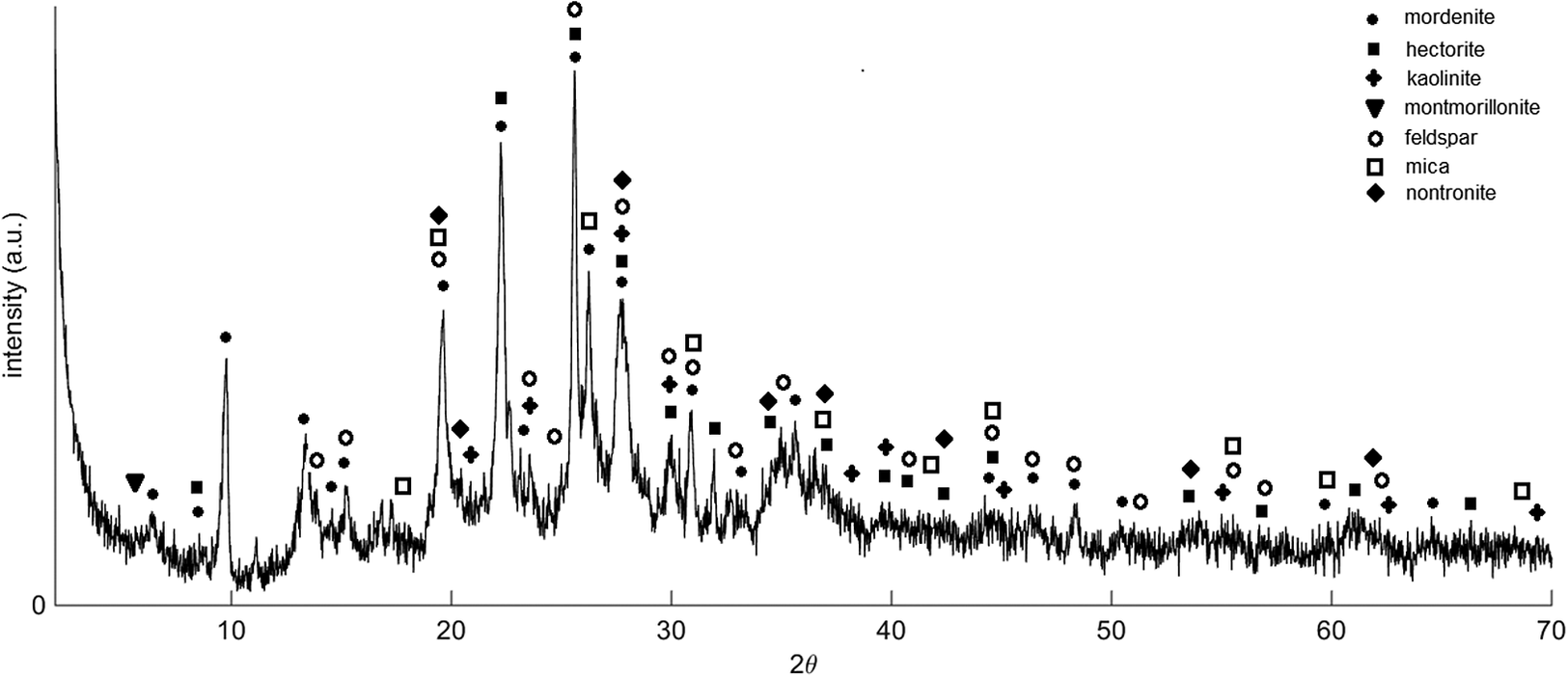
Figure 1. X-ray diffractogram of the raw Philippine natural bentonite.
4.2. Chemical analysis
Various chemical analyses such as gravimetric, titration, and flame-atomic absorption spectrometry were done to verify and determine the components of the raw Philippine bentonite. The chemical composition, according to the supplier’s chemical analysis report from an independent laboratory (Chemistry Laboratory of the Mines and Geosciences Bureau, Department of Environment and Natural Resources, Philippines) is given in Table 1. The most abundant components were silica and alumina which comprise 47.90% and 14.02% of the total weight, respectively. It is worth mentioning that these two components determine the number of Si and Al present in the bentonite. Si/Al ratio influences the efficiency of the material to adsorb cation or its CEC (Gili & Conato, Reference Gili and Conato2019). Normally, the lower the Si/Al ratio, the higher the maximum adsorption capacity, the greater the CEC. This means that the material might be a good adsorbent of heavy metals and other positively charged molecules as one of its many applications. It was then followed by ferric oxide which accounts for 7.54% and lime which is 4.83% of the total weight of the powder sample. MgO accounts for 1.59% of the total weight while K2O and Na2O are 0.46% and 0.33%, respectively. The powder sample absorbs a considerable amount of moisture at 10.71 weight%. The loss of ignition is around 12.27%. This might probably be attributed to the volatile materials present in the sample such as organic matter which burns at elevated temperatures.
Table 1. Chemical components of the Philippine bentonite based on chemical tests
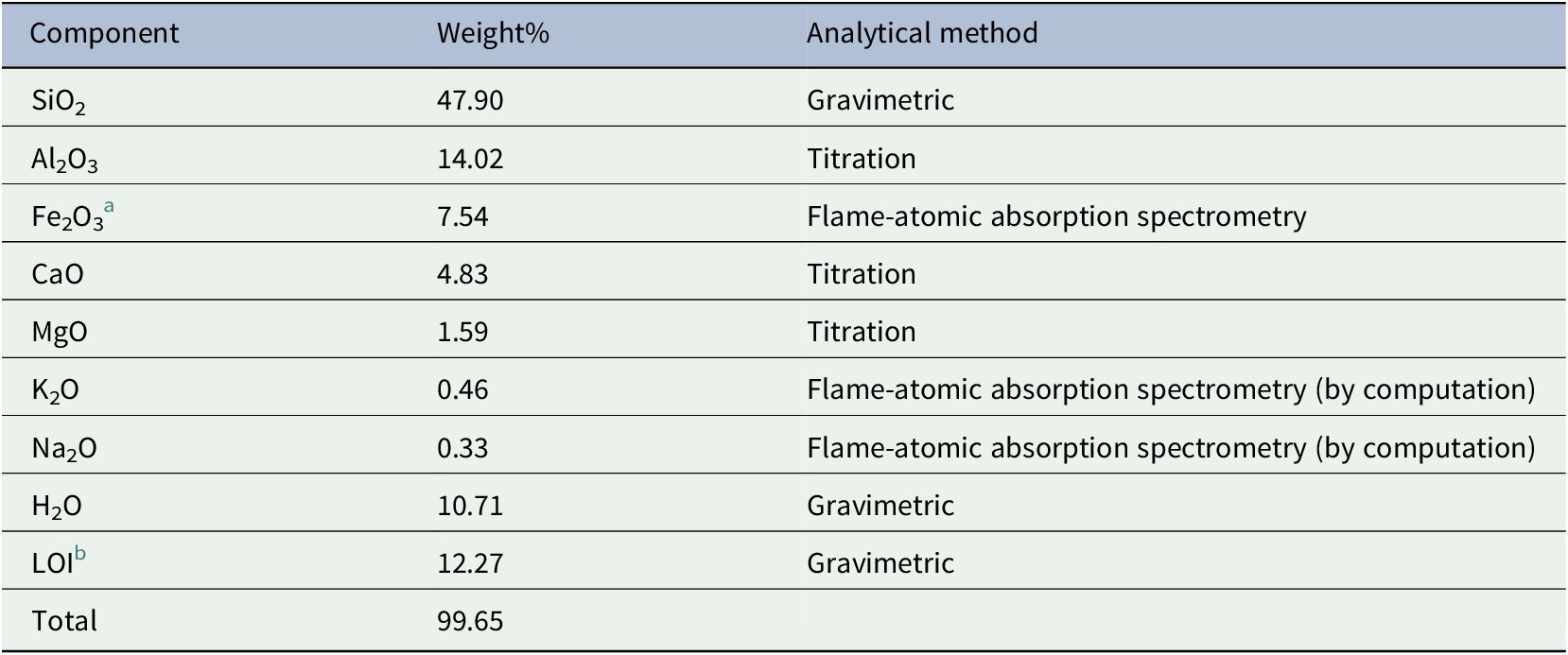
a Computed from Feγ.
b Loss on ignition.
4.3. Scanning electron microscopy
Figure 2 shows the SEM images of the raw Philippine natural bentonite. The powder samples are composed of particles of irregular size and shapes. Some particles agglomerate creating bigger particles. However, most particles are less than 50 μm in size. At higher magnification, the powder is made up of particles with flaky and porous textures. The flaky and porous texture is advantageous as it gives the material a higher surface area which means higher chances of interaction for cation exchange and absorption purposes.
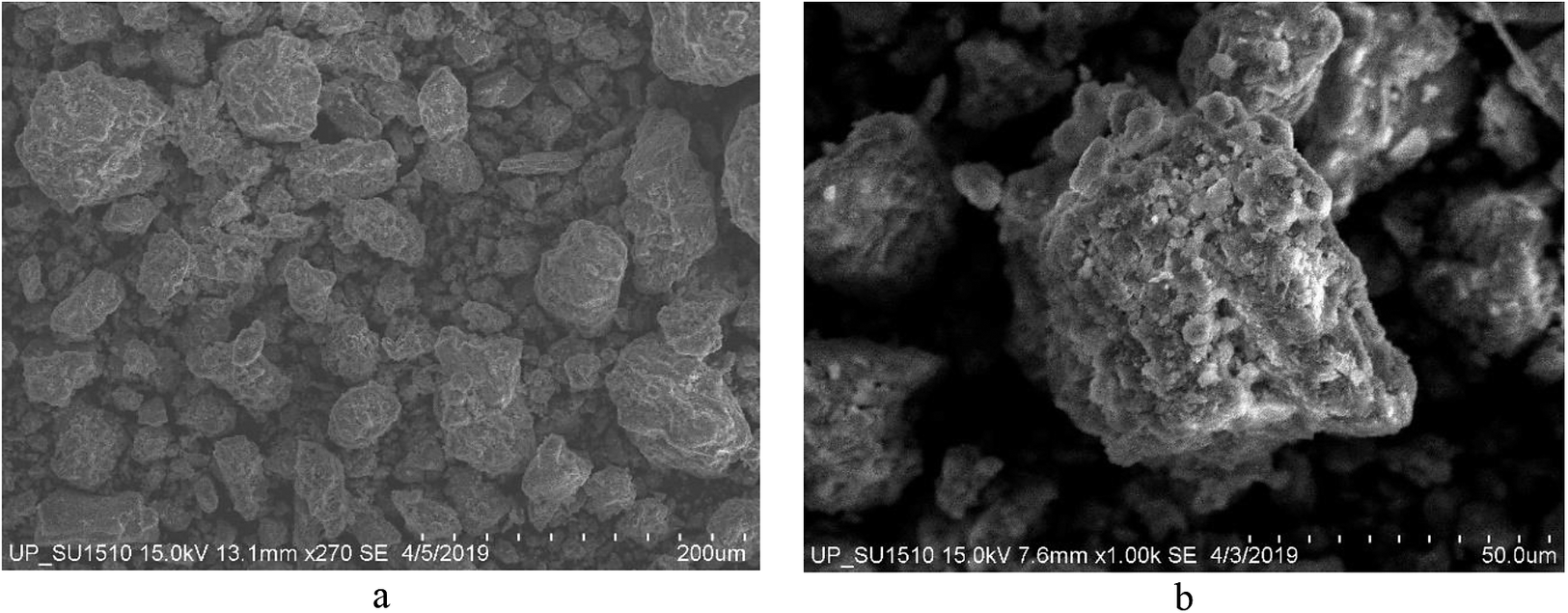
Figure 2. Scanning electron microscopy (SEM) images of the Philippine natural bentonite at different magnifications: (a) 270× and (b) 1,000×.
4.4. Thermogravimetric-differential scanning calorimetry
Figure 3 shows the TG-DSC curve of the Philippine bentonite. Three notable weight loss was recorded. The first one is a substantial weight loss occurring from room temperature up to 240°C. The weight loss is equal to 15.55% which is attributed to the moisture content of the bentonite. The endothermic peak (minima) is centered at around 100°C which is the vaporization point of water. The second reduction in weight occurs at 240°C up to 500°C which recorded a further loss in weight of 3.51%. This can be accounted to the evaporation of tightly bound water in the zeolitic components of the mineral (Olegario et al., Reference Olegario, Felizco and Mulimbayan2017). Note that there is another endothermic peak at 485°C suggesting dehydroxylation of hydroxyl functional groups. Dehydroxylation involves the heating process through which the hydroxyl group (OH) is released by forming a water molecule (Frost & Vassallo, Reference Frost and Vassallo1996). Finally, a weight loss of 2.13% which occurred at 500–1,000°C is due to the release of volatile materials. In total, the loss in weight of the bentonite after heating at 1,000°C is equal to 21.19% of the initial mass. Comparably, the thermogravimetric curve of the Philippine bentonite is almost the same as that of Philippine natural zeolite, which recorded an approximately 17% decrease in mass after heating at 1,000°C (Olegario et al., Reference Olegario, Felizco and Mulimbayan2017). The two minerals are mined from the same site in Mangatarem, Pangasinan in Luzon Island, Philippines.

Figure 3. Thermogravimetric-differential scanning calorimetry curve of the Philippine bentonite.
4.5. Fourier transform infrared analysis
Figure 4 shows the infrared (IR) transmittance spectrum of the Philippine natural bentonite. The vibration bands present in the sample are as follows: the band at 3,622 cm−1 corresponds to the O–H stretching of inter-porous water (Osonio & Velasquez, Reference Osonio and Velasquez2018). The vibration bands at 3,392 and 2,987 cm−1 correspond to the symmetric and asymmetric stretching of hydroxyl functional groups (Prasetyp & Soegijono, Reference Prasetyp and Soegijono2018). The bands at 1,632 and 1,414 cm−1 are attributed to the typical bending of H2O molecules attached to the surface of the zeolite (Karge, Reference Karge2001; Osonio & Velasquez, Reference Osonio and Velasquez2018), whereas 1,205, 1,000, 913, and perhaps 876 cm−1 are due to the asymmetrical stretching of internal tetrahedra (O–Si–O and O–Al–O; Osonio & Velasquez, Reference Osonio and Velasquez2018). The vibration band at 792 cm−1 is assigned to the symmetrical stretching of external linkages while the vibration groups at 748 and 688 cm−1 are due to the symmetrical stretching of internal tetrahedra. Note that the bands in the 600–800 cm−1 range are assigned to exchangeable cations (Olegario et al., Reference Olegario, Felizco and Mulimbayan2017).
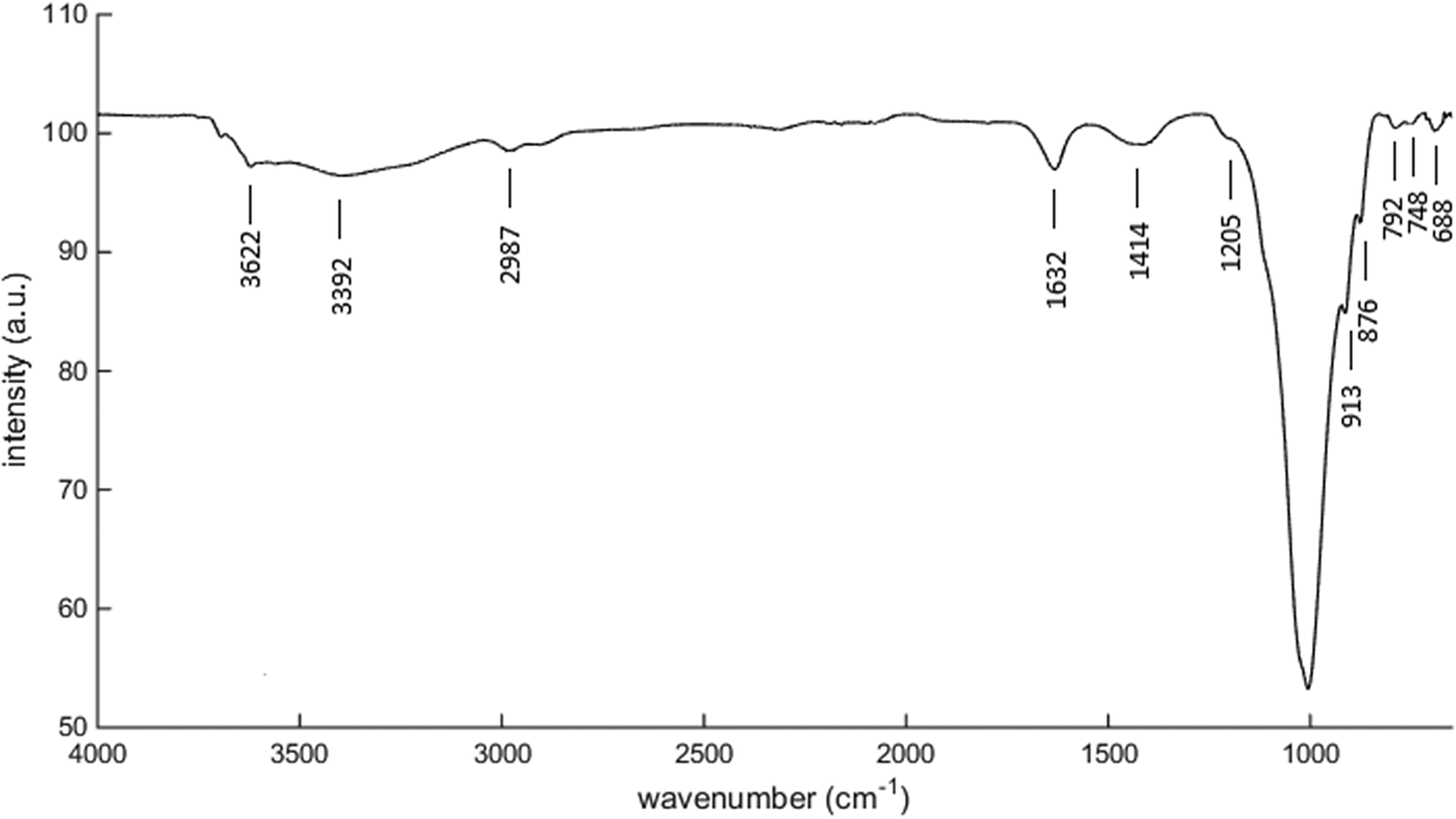
Figure 4. Infrared (IR) transmittance spectrum of raw Philippine natural bentonite.
4.6. Cation exchange capacity
The Philippine natural bentonite was tested for CEC. According to the result of an independent experiment conducted by Lipa Quality Control Center, 001 J.P. Laurel National Highway, Purok 4, Brgy. Sico, Lipa City, Batangas 4,217, Philippines, the CEC of the bentonite is 91.37 meq/100 g using the ammonium acetate method. On the other hand, the measured CEC according to the barium acetate method is 43.01 meq/100 g. There are several factors on why the two values are not equal. The disadvantage of the Ba(OAc)2 method seems to be associated with the low buffer capacity of the solution such that exchange acidity is not displaced (Pratt & Holowaychuk, Reference Pratt and Holowaychuk1954). Ba(OAc)2 method was inefficient in the displacement of H ions (Hanna & Reed, Reference Hanna and Reed1948). The low values for the Ba(OAc)2 procedure is probably more associated with exchangeable Al than with exchangeable H (Pratt & Holowaychuk, Reference Pratt and Holowaychuk1954). The measured CEC values are much higher than natural clinoptilolite and other selected materials (Gili, Olegario-Sanchez, & Conato, Reference Gili, Olegario-Sanchez and Conato2019). This means that the natural bentonite is a good candidate adsorbent for the sorption of heavy metals and other cationic pollutants in an aqueous environment.
5. Conclusions
The Philippine natural bentonite is composed primarily of mordenite, hectorite, and montmorillonite. Traces of kaolinite, feldspar, mica, and nontronite are also present in the clay mineral. It was found that the Philippine bentonite is composed mainly of silica (47.90 wt%) and alumina (14.02 wt%) according to chemical analysis. SEM images reveal that the powder is made of particles with flaky and porous morphology. TG-DSC shows that the bentonite contains a significant amount of moisture amounting to 15.55 wt% excluding the tightly bound water which accounts for 3.51% of the total weight of the bentonite. IR transmittance spectrum of the bentonite sample shows the common vibration bands present in bentonite samples. It was found that the CEC of the Philippine natural bentonite is 91.37 and 43.01 meq/100 g according to ammonium acetate and barium acetate methods, respectively.
Acknowledgments
The authors would like to thank Dr. Tsumotu Sato of the Environment Geology Laboratory under the Division of Sustainable Resources Engineering in Hokkaido University for the use of analytical equipment for the mineralogy and morphology of the bentonite minerals.
Authorship Contributions
M.Z.G. conceived and designed the study. M.Z.G. and E.M.O. conducted data collection, analysis, and interpretation. Both authors contributed to writing the article.
Funding Statement
This research was not funded by any agency, organization, or institution. However, the authors would like to thank the Applied Physics Research Section of the Philippine Nuclear Research Institute for its support in the conduct of the study.
Conflicts of Interest
The authors have no conflicts of interest to declare.
Data Availability Statement
The data that support these findings are openly available upon request to the corresponding author.
Comments
Comments to the Author: This paper describes the characterization of Philippine natural bentonite.
The topic is important in clay researcher. Other physical properties such as colour, density, hardness (Moh’s scale), calcination temperature should be added.
Regarding references: there are 2 references for 2018, 3 references for 2019 and one for 2020. These references will have merit to be presented.