Introduction
Olive groves play a fundamental role in the production system of the southern regions of Europe. In 2020, the gross value of olive production was 6762 million US$ in Spain, 19,349 in Europe and 23,891 worldwide (FAOSTAT, 2022).
Most of these earnings come from traditional olive groves. This type of olive grove has long been considered a typical Mediterranean agroecosystem of great importance in the maintenance of biodiversity and the control of soil erosion, and as part of the ancient Mediterranean cultural heritage and landscape identity (Loumou and Giourga, Reference Loumou and Giourga2003).
However, the traditional olive grove, like other Mediterranean agroecosystems, is currently undergoing dramatic changes due to intensification, changes in soil management techniques and, in many cases, abandonment. All these processes are being mediated by globalization (Guzmán-Álvarez and Navarro-Cerrillo, Reference Guzmán-Álvarez and Navarro-Cerrillo2008; Lozano-García et al., Reference Lozano-García, Muñoz-Rojas and Parras-Alcántara2017). These changes are having significant effects on the ecosystem services provided by olive farming and on crop sustainability. In recent times, new intensive olive groves have been planted in areas where cereals or traditional olive groves once dominated. This, combined with the progressive increase in production costs, the increasing average age of farmers, and the lower value of the olives and olive oil, are making traditional olive groves less profitable. Sloping olive groves are particularly vulnerable to changes of this kind (Duarte et al., Reference Duarte, Jones and Fleskens2008) because mechanization of farm work is limited by problems of accessibility and drivability of agricultural machinery on steep hillsides (Blanco, Reference Blanco2009; CAPDR, 2018).
In Spain, olive groves cover a total area of 2,770,424 ha., of which 1,673,657 ha are in Andalusia (ESYRCE, 2021). There are adult plantations of olives on surfaces with many different degrees of slope. 29.6% of Andalusian olive groves are on slopes of over 20%, the threshold above which total mechanization is considered impossible (Blanco, Reference Blanco2009; CAPDR, 2018). With the current trend toward mechanization, these olive groves are becoming less and less viable and are therefore at serious risk of being abandoned. Surveys on land use (ESYRCE, 2021) indicate that the percentage of ‘unmaintained’ olive groves in Spain is 6.6%, and 3.4% in Andalusia. Many of these ‘unmaintained’ olive groves may in fact have been abandoned. That makes it difficult to quantify the ‘real’ area of abandoned olive groves and how this has changed over time.
Traditional olive groves are based on a conventional farming system in which the soil is tilled to control ground cover. For the last several decades, tillage has been complemented by the use of inorganic fertilizers and synthetic pesticides. Tillage is especially detrimental in strongly sloping areas, where it can lead to severe soil erosion. That is why soil management must be taken into account when assessing the environmental services provided by agroecosystems, in which it can be just as or even more important than the farming system applied (Soriano et al., Reference Soriano, Álvarez, Landa and Gómez2014; Sánchez-Fernández et al., Reference Sánchez-Fernández, Vílchez-Vivanco, Navarro and Castro-Rodríguez2020).
On the other hand, although little research has so far been conducted into what happens to olive groves once they have been abandoned, it seems that the flora undergo a rapid secondary succession process, and a phenomenon known as ‘renaturalization’ or rewilding (Rühl et al., Reference Rühl, Caruso, Giucastro and La Mantia2011) occurs, as happens in other types of agricultural land, in which pastures are progressively transformed into scrublands, shrub formations and later into woodland (di Pietro and Blasi, Reference di Pietro and Blasi2002; Loumou and Giourga, Reference Loumou and Giourga2003; Rühl et al., Reference Rühl, Caruso, Giucastro and La Mantia2011; Maccherini et al., Reference Maccherini, Santi, Bonini, Amici, Pruscini, Palazzo and Selva2013; Romero-Díaz et al., Reference Romero-Díaz, Ruiz-Sinoga, Robledano-Aymerich, Brevik and Cerdà2016). The herbaceous flora in the early grassland and scrub seasons show high diversity and are dominated by Poaceae and Fabaceae species (Lasanta et al., Reference Lasanta, Sánchez-Navarrete, Medrano-Moreno, Khorchani and Nadal-Romero2020). This process depends on the time elapsed since the grove was abandoned (García, et al., Reference García, Tarrasón, Mayol, Male-Bascompte and Riba2007; Solomou and Sfougaris, Reference Solomou and Sfougaris2015), as well as on flora composition and the soil seed bank. Other important factors include the plant communities that surround it (Solomou and Sfougaris, Reference Solomou and Sfougaris2015; Rey et al., Reference Rey, Manzaneda, Valera, Alcántara, Tarifa, Isla, Molina-Pardo, Calvo, Salido, Gutiérrez and Ruiz2019) and any isolated trees within the olive grove which can act as perches (Rühl et al., Reference Rühl, Caruso, Giucastro and La Mantia2011). Rewilding is also affected by edaphic issues, stoniness, slope, soil penetration resistance, the presence of terraces (García et al., Reference García, Tarrasón, Mayol, Male-Bascompte and Riba2007; Maccherini et al., Reference Maccherini, Santi, Bonini, Amici, Pruscini, Palazzo and Selva2013; Perrino et al., Reference Perrino, Ladisa and Calabrese2014), overgrazing -which prevents succession and encourages erosion- (Arhonditsis et al., Reference Arhonditsis, Giourga and Loumou2000; Maccherini et al., Reference Maccherini, Santi, Bonini, Amici, Pruscini, Palazzo and Selva2013), and fires (Rühl, et al., Reference Rühl, Caruso, Giucastro and La Mantia2011).
In order to understand more about the patterns and processes affecting plant diversity (as an indicator of biodiversity) in sloping olive groves at high risk of abandonment and how they are affected by farming system (conventional-organic-abandonment) and soil management (native cover crop vs tillage), 20 different locations were studied across Andalusia. The ultimate goal is to provide data that will be useful for taking decisions about the future of sloping olive groves in line with the European Green Deal and the Biodiversity Strategy for 2030. We predict that the way an olive grove is managed will be reflected in the biodiversity. The following hypotheses were tested: (i) organic farming and abandonment will provide more biodiversity than conventional farming; (ii) soil management practices such as native cover crops will also have a significant influence on biodiversity, although it will be strongly mediated by environmental variables such as climate, soil features or landscape structure.
Material and methods
Study site
We selected 20 locations with sloping olive groves situated on slopes with a gradient of over 20% within a total area of 1 million ha in the provinces of Granada, Jaén, Córdoba and Málaga (Andalusia, Spain) (Fig. 1). Three adjacent or very close plots were selected in each locality, one from each of the three farming systems: organic (O), conventional (C) and abandoned (A). They were all mature olive groves, with more than one trunk per tree, an average age of 55 years, from 100–139 trees ha−1 and a canopy volume ranging between 1019–5350 m3 ha−1 (Table 1). We contacted the owners of the plots to find out about the farming systems used and how long the abandoned groves had been in that condition. We then classified each plot according to the farming system and soil management technique applied (Pastor et al., Reference Pastor, Castro, Vega, Humanes, Barranco, Fernández-Escobar and Rallo2004; ESYRCE, 2021, see Fig. 2), as follows:
• Organic Tillage (OT): certified-organic management with soil tillage (n = 4).
• Organic Cover Crops (OC): certified-organic management with a cover crop controlled by mowing (n = 16).
• Conventional Tillage (CT): traditional soil tillage. Depending on the intensity of the tillage, soil can have some weed cover in autumn and early spring (n = 11).
• Conventional Non-Tillage (CNT): application of pre- and post-emergence herbicides over the soil surface. Some later-emerging weeds may escape the effects of the herbicides (n = 9).
• Abandoned Cover Crops (AC): Sloping olive groves abandoned between 2000 and 2015, with herbaceous vegetation covering the soil surface (i.e., first stages of abandonment) (n = 5).
• Abandoned Woody (AW): Sloping olive groves abandoned between 1950 and 2000, with woody vegetation established by secondary succession (i.e., consolidated abandonment) (n = 15).
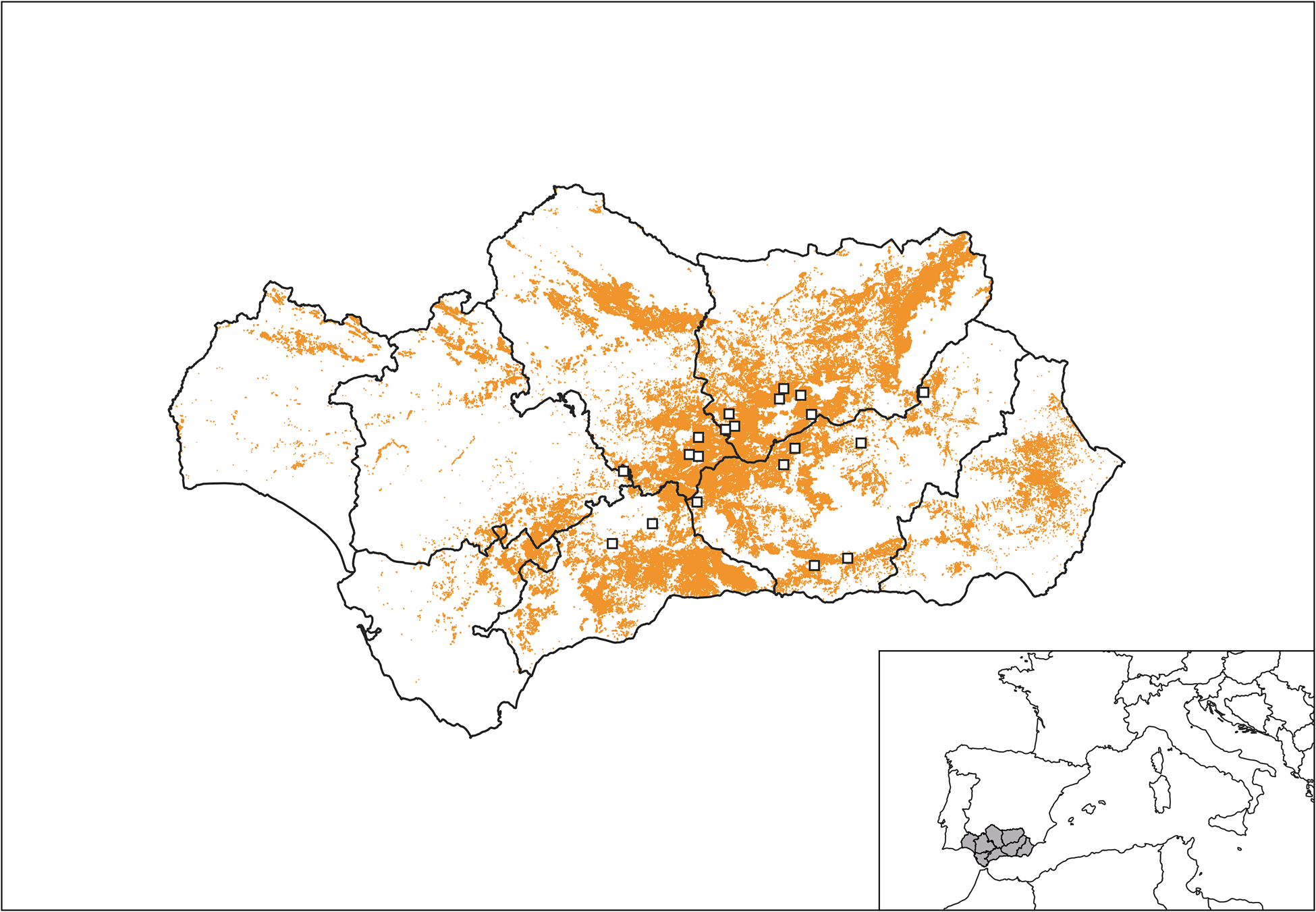
Fig. 1. Location of the study places (□) in Andalusia (South Spain). In orange distribution of olive groves with slope > 20%.

Fig. 2. Combinations of farming system and soil management analyzed in this study.
Table 1. Information about the localities studied, including mean data recorded at plot level for geographical coordinates (X, Y), slope, mean air temperature (Tavg), mean annual precipitation (Pavg), altitude, potential insolation, canopy volume, soil coverage tree canopy, tree mound, years of abandonment (applicable for abandoned plots), olive tree age, soil shear stress, pH, bulk density, soil organic carbon, soil nitrogen, C:N ratio, soil potassium, cation exchange capacity, gravels, clay, water resource depletion and variables measured at 1-km buffer cover surface area (ha) around the plots

In the treatments involving tillage, this was done twice a year, in the autumn and the spring. In these cases, plant sampling was always carried out before the spring tillage. In all cases, the same soil management technique was applied in each plot from at least 2009 onwards. When we use the term ‘cover crops’, we are referring to native or spontaneous cover crops.
Plant sampling
Plant sampling was carried out during spring 2017. Data about the vascular plants were recorded through three line transects of 30 m in length, with a bar perpendicularly touching every 1 m of the measuring tape. Transects were always arranged perpendicular to the slope. The selection of the starting point of each transect inside the plots was randomly selected. Starting at 0.5 m, 30 points per transect and 90 per plot were monitored using an adapted version of the point-quadrat sampling technique (Chalmers et al., Reference Chalmers and Parker1989). All the species from the different layers touching the bar at the same point were registered. Each vascular plant was counted only once, even if the bar touched the same individual several times. Bare ground, litter, gravels (rock <4 mm), rock (rock >4 mm) and bryophytes were recorded when no plants were found. The contacts with the canopy of the olive tree were also counted. From these data, we calculated the total and partitioned cover (%) of both vascular plant species and the other surface layers (litter, gravels, rocks, bryophytes, bare ground).
From the floristic data we estimated plant species richness (S), i.e., the total number of species per sample; abundance (N), i.e., the number of individuals of each species per sample; and species diversity calculated from the above using the Shannon–Wiener (H’), Simpson (D) and Margalef indexes (Magurran, Reference Magurran1988). The Shannon–Wiener index measures the uncertainty of a category in a particular set. The Simpson index represents the probability that two randomly selected individuals from the habitat will belong to the same species, and the Margalef index provides a measure of species richness that is roughly normalized for sample size without using more complex rarefaction techniques. These indexes are widely used in studies of plant species and composition in olive groves and higher index values mean higher diversity (Solomou and Sfougaris, Reference Solomou and Sfougaris2011, Reference Solomou and Sfougaris2013, Reference Solomou and Sfougaris2015; Al Harbi, Reference Al Harbi2017; Gómez et al., Reference Gómez, Campos, Guzmán, Castillo-Llanque, Vanwalleghem, Lora and Giráldez2018; Carpio et al., Reference Carpio, Lora, Martín-Consuegra, Sánchez-Cuesta, Tortosa and Castro2020) The species abundance by plant families, Raunkiaer life forms (therophytes, geophytes, hemicryptophytes, chamaephytes, nanophanerophytes and phanerophytes), life cycle (annual and perennial) and distribution range were also analyzed. The methods for identifying species and families, life form, life cycle and distribution were based on Castroviejo (Reference Castroviejo1986), and Blanca et al. (Reference Blanca, Cabezudo, Cueto, Fernández López and Morales Torres2009).
Environmental data
We recorded geographic and topographic variables (latitude, altitude, slope), climatic variables (mean annual temperature and precipitation, potential insolation), soil features (shear stress, soil organic carbon, N, P and K contents, C:N ratio, pH, cation exchange capacity, gravel content, clay content and water resource depletion), tree status at plot level (soil coverage at tree level, olive tree age and years since abandonment), and data registered at landscape level (mean data recorded at 1-km buffer around the plots to analyze landscape complexity and cover surface areas of olive groves, shrubland, grasslands, broadleaf-forest, conifer, rainfed cereals, woody-crop, water-body and artificial surface).
Climate date such as altitude (m), mean annual air temperature (°C), mean annual precipitation (mm) and potential insolation (total hours of sunlight per year) were extrapolated from REDIAM (2019), while the slope (%) was measured with a clinometer. Tree age and year since abandonment were obtained directly from land owners and Orthoimages of REDIAM (2019). Trunk perimeter (at 30 cm above the soil) and height was measured manually, and canopy volume (m3_ha−1) and tree cover (m2 ha−1) were calculated from tree height and spread according to Gálvez et al. (Reference Gálvez, Parra and Navarro2004). Tree mound (soil loss around trunk, m3_ha−1) was obtained using the Stocking and Murnaghan (Reference Stocking and Murnaghan2001) soil degradation index.
Soil bulk density (g_cm3) was calculated according to Grossman and Reinsch (Reference Grossman, Reinsch, Dane and Clarke Topp2002), and soil parameters such as gravels (%), clay (%), pH, CEC (cmol+_kg−1), soil phosphorus (Mg_ha−1), soil potassium (kg_ha−1) and water resource depletion (mm) according to MAPA (1986). The C:N ratio was also analyzed to a soil depth of 30 cm. C was analyzed by wet oxidation with the dichromate method (Nelson and Sommers, Reference Nelson, Sommers and Sparks1996), and N using the Kjeldahl method (Bremner, Reference Bremner and Sparks1996).
At landscape level we established a buffer of 1-km radius around the centroid of each sampling plot following the methodology of Scalercio et al. (Reference Scalercio, Brandmayr, Iannotta, Petacchi and Boccaccio2012) to analyze the vegetation cover and land use based on the Andalusian Map of Land Uses and Vegetation Covers from 2017 (E 1:25,000; REDIAM, 2019). We obtained 55 different land uses and vegetation covers, which were then grouped into these broad categories: olive groves, shrubland, woody crops, broadleaf forests, grasslands, conifers, rainfed cereals, water bodies and wetlands and artificial surface. On the basis of the number of categories found in each buffer and the number of different patches from each category, we then measured landscape complexity using the Shannon index.
Data analysis
The diversity of vascular plants was estimated through the number of species, the number of individuals from each species, and the diversity indexes in order to determine the specific structure and richness of the population of plant species and to analyze the effects of the different treatments (Magurran, Reference Magurran1988). For this purpose, one-way ANOVAs or Kruskal–Wallis tests were applied with treatments as the independent variable. Normality and homogeneity of variance tests were carried out, after which one-way ANOVAs were applied to the biodiversity-related parameters, and Kruskal–Wallis tests were applied to soil covers.
To analyze the effects of the different treatments on the whole species composition, we used cluster analysis in Q-mode and UPGMA (unweighted pair-group method using the arithmetic average based on the Bray–Curtis similarity index). For this purpose, we used the presence-absence of species and aggregated the data by treatments.
A dissimilarity analysis (ANOSIM), which was also based on the Bray–Curtis similarity index (Clarke, Reference Clarke1993), was performed as a non-parametric test that allows significant differences between two or more groups to be identified. The R-test statistic expresses dissimilarity between the groups, over a range of 0 to 1. When R values are close to or equal to 1 and P ⩽ 0.05, this is a sign of significant dissimilarity (Legendre and Legendre, Reference Legendre and Legendre1998). This analysis was carried out for species, family, chorology and life form for all the treatments analyzed globally and by pairs of treatments.
We also carried out a SIMPER analysis (percentage similarity procedure; Clarke, Reference Clarke1993) on the pairs that showed significant differences in ANOSIM. Species, family, chorology and life form were also analyzed.
The effect of the environmental variables was analyzed via redundancy analysis (RDA), a constrained ordination method proposed by Ter Braak and Smilauer (Reference Ter Braak and Smilauer2002) for linear data, which was verified by the length of the gradient of the first axis (<2) of a Detrended Correspondence Analysis (DCA) made with the species matrix. The individual significance of the environmental variables was analyzed through the marginal effects (ʎ1) obtained using automatic forward selection, although the variables finally included in the RDA were those showing higher conditional effects (ʎA) and P ⩽ 0.05 (the effect contributed by each variable over and above the combined effect of all the other variables already selected, so avoiding collinearity). The variability in the species and samples due to environmental variables was tested using the Monte Carlo permutation test (499 permutations) for the first and all canonical axes.
Index calculation and statistical analysis were performed on the data using Past software ver. 3.2 (Hammer et al., Reference Hammer, Harper and Ryan2001), and the Statistix 9.0 software package. Multivariate analyses (DCA, RDA) were carried out using CANOCO 4.5 (Analytical Software, Tallahassee, FL, USA) (Lepš and Šmilauer, Reference Lepš and Šmilauer2003).
Results
The OC treatment showed the highest mean cover of vascular plant species (54.5% ± 1.8 SE), especially compared to the treatments involving tillage (OT = 23.1% ± 5.4, and CT = 18.1% ± 3.4). Bare ground also obtained higher values in these last two variables than OC, AC and AW, while CNT obtained intermediate values (Table 2). Litter cover was minimal in the tilled treatments, while it was higher in the abandoned olive groves (AW and AC) and in organically farmed groves with a native cover crop (OC). Bryophyte cover was significantly higher in the abandoned olive groves (AC and AW) while in the treatments involving tillage, either no bryophytes were found (OT) or the value was very low (CT). Gravels and rocks on the surface of the soil were particularly high in AW and CNT (Table 2).
Table 2. Mean percentage (%) ± standard error (SE) for the area covered by different soil covers in each treatment

OT, organic tillage; OC, organic cover crops; CT, conventional tillage; CNT, conventional non-tillage; AC, abandoned cover crops; AW, abandoned woody.
The Kruskal–Wallis test was performed at P ⩽ 0.05. Different letters show significant differences in the Kruskal–Wallis All-Pairwise Comparisons Test. The last column shows the mean for each soil cover.
A total of 294 species from 45 plant families were accounted for in this study. For most of the treatments analyzed, the flora was dominated by annual species ranging from 84% in CT to 91% in OC, with the exception of AW olive groves, where perennial species dominated with 53% (Supplementary Table S1).
At plot level, the highest mean species richness was found in OC (27.9 ± 3.0), AC (21.2 ± 3.7) and AW (27.2 ± 3.0), and the lowest in OT (10.0 ± 5.4) and CT (8.1 ± 2.2) (Table 3). The highest individual abundance was also found in OC (146.6 ± 17.9) and the lowest in CT (25.8 ± 10.4) and OT (21.8 ± 14.1). CT obtained the lowest values in both the Simpson index and the Shannon index (0.63 ± 0.1; 1.4 ± 0.3 respectively). These values were significantly different from the highest values, which were obtained by OC, AC and AW. In general, CNT obtained intermediate values while the lowest values were obtained by the treatments involving tillage (Table 3). The treatments involving tillage (OT and CT) showed significantly lower values on the Margalef index, while AW scored the highest value (5.7 ± 0.5).
Table 3. Mean values ± standard error of species richness (S), abundance (N) and diversity indices

OT, organic tillage; OC, organic cover crops; CT, conventional tillage; CNT, conventional non-tillage; AC, abandoned cover crops; AW, abandoned woody.
Different letters denote Tukey's HSD post hoc significant differences (P ⩽ 0.05) for the treatment factor in each parameter (one-way ANOVA).
1 Values followed by different letters in the same row show significant differences at levels: NS = No Signif.; *P ⩽ 0.05; ** P ⩽ 0.01; *** P ⩽ 0.001.
2 # Transf. log(x).
Cluster analysis separated the combinations involving tillage (CT and OT) from all the others (Fig. 3). Of these, AW and OC were most similar to each other and were different from the cluster formed by CNT and AC.

Fig. 3. Hierarchical clustering using the Bray–Curtis similarity index and cluster analysis in Q-mode and UPGMA for each treatment. OT = organic tillage; OC = organic cover crops; CT = conventional tillage; CNT = conventional non-tillage; AC = abandoned cover crops; AW = abandoned woody.
The analysis of similarity (ANOSIM) for plant species composition, plant family, chorology and life form based on abundance data was statistically significant when globally analyzed (Table 4). Analyzed by pairs of treatments, AW showed significant P values for all pairwise comparisons, showing the highest R (dissimilarity) values when compared to the tilled treatments (especially OT). In all the variables studied, significant P values were obtained in the comparison between OC and OT and the highest R values. In general, at species and family composition level, OT was different from all the other treatments except CT and CNT. As regards chorological distribution and life form, OC and AW showed dissimilarity from all the other treatments, and the highest R value (0.7) was obtained in the AW-OT pair for life form (Table 4) (Supplementary Table S2).
Table 4. Analysis of similarities (ANOSIM) for species, family, chorology and biotype both globally for all treatments and for pairs of treatments

OT, organic tillage; OC, organic cover crops; CT, conventional tillage; CNT, conventional non-tillage; AC, abandoned cover crops; AW, abandoned woody.
Only the R values that showed significance at a level of P ⩽ 0.05 are shown.
According to the SIMPER analysis (Table 5), the dominant life form was the therophyte (with a 64.8% contribution to dissimilarity), which was most abundant in OC (44.3). By contrast, AW showed high abundance values for chamaephytes, hemicrytophytes and nanofanerophytes. The main families contributing to the dissimilarity between the treatments were Poaceae, Fabaceae and Asteraceae (64%). Poaceae species were especially abundant in OC, AC and to a lesser extent AW, while Fabaceae and Asteraceae species were most abundant in OC. The abundance of Fabaceae species was especially low in the tilled olive groves (OT and CT). CT was the only treatment with an abundance of Fumariaceae and Papaveraceae species, while AW had the greatest abundance of Lamiaceae, Cistaceae, Rhamnaceae, Fagaceae (Quercus species), and typical families of geophytes such as Orchidaceae, Iridaceae and Liliaceae, which were not found in the other treatments. According to this analysis, the Mediterranean, Iberian and Paleartic species were the ones that encompassed most of the dissimilarity between the treatments (81.7%). Of these, the Mediterranean species make the greatest contribution to dissimilarity (56.7%) and were dominant in all the treatments, especially OC, while the Iberian and Baetic species dominated in AW (Supplementary Table S2).
Table 5. SIMPER analysis for biotypes, families and chorological types

OT, organic tillage; OC, organic cover crops; CT, conventional tillage; CNT, conventional non-tillage; AC, abandoned cover crops; AW, abandoned woody.
The dissimilarity average (Bray–Curtis distance), the contribution of each type, and the accumulated contribution are presented. The highest values that contribute most to the differentiation appear in shading.
The contribution of the main plant species to dissimilarity (up to 68.5%) was especially notable in OC and AW (Table 6). Annual grasses and legumes were dominant in OC. AW had a quite different plant species composition, with higher abundance of perennial and woody species (Supplementary Table S2), while the treatments involving tillage were dominated by ruderal species such as Lolium rigidum, Diplotaxis virgata and Eruca vesicaria.
Table 6. Results of SIMPER analysis showing the dissimilarity average (Bray–Curtis distance), and the contribution (%) made by the different plant species (total, cumulative and for each treatment).

OT, organic tillage; OC, organic cover crops; CT, conventional tillage; CNT, conventional non-tillage; AC, abandoned cover crops; AW, abandoned woody.
The highest values that contribute most to the differentiation appear in shading. The cumulative contribution (%) made by all these species comes to 68.5%.
Species richness (S) was also influenced by a range of different environmental variables. In particular, it was highly and positively correlated with soil organic carbon content (r = 0.617, P = 0.000), soil nitrogen content (r = 0.516, P = 0.000), soil shear stress (r = 0.404, P = 0.001), and to a lesser extent with soil gravel percentage, cation exchange capacity, C:N ratio, age of the olive grove, and mean annual precipitation (Table 7). By contrast, species richness was negatively correlated with the presence of woody crops (olive groves) and the area of rainfed cereal calculated at landscape level, soil pH, water resource depletion, olive canopy volume, mean annual temperature and tree mound (Table 7). Abundance (N) was also highly correlated with the soil organic carbon (r = 0.757, P = 0.000), nitrogen (r = 0.684, P = 0.000), potassium and phosphorus contents and with cation exchange capacity (Table 7), as well as with soil shear stress and mean annual precipitation. It was negatively correlated with soil pH (r = −0.461, P = 0.000), and with the presence of patches of woody crops (olives) at landscape level.
Table 7. Pearson's correlation coefficients between soil/agro-environmental variables with abundance of individuals (N) and species richness (S). The asterisks show significant differences at 0.05 (*); 0.01 (**); and 0.001 (***).

RDA conducted using the abundance of individuals by plant families clearly segregated the conventional combinations (C) from the organic combinations (O) and the abandoned olive groves (A). More specifically, the AW and OC combinations showed a higher abundance of individuals belonging to families such as Fabaceae, Poaceae and Asteraceae, etc., while families such as Fumariaceae, Polygonaceae and Papaveraceae were more abundant in OT and CT (Fig. 4). AC and CNT appeared in an intermediate position between OC and OT-CT. The effects of the explanatory variables (Monte Carlo test with 499 permutations) were highly significant for the first canonical axis (Eigenvalue = 0.166, F-ratio = 9.744, P = 0.002) and for all the canonical axes (Trace = 0.339, F-ratio = 2.509, P = 0.002). The total variance explained by all the variables used in the RDA was 69.6%. The main variables explaining the total variance in the data regardless of their interactions (singly analyzed, marginal effects) were soil organic carbon and nitrogen, soil shear, mean annual temperature and precipitation and olive age. When these effects were taken into account (conditional effects), the significant variables were soil organic carbon (λ = 0.11, F = 7.44, P = 0.002), soil phosphorous content (λ = 0.04, F = 2.53, P = 0.010), soil shear (λ = 0.03, F = 2.17, P = 0.018), mean annual temperature (λ = 0.04, F = 2.38, P = 0.010), slope (λ = 0.03, F = 2.24, P = 0.006), artificial surface (λ = 0.02, F = 1.69, P = 0.052) and soil clay (λ = 0.02, F = 1.67, P = 0.040). In addition to having a higher abundance of individuals belonging to the main plant families, both OC and AW were influenced by and positively correlated with the soil organic carbon, nitrogen and phosphorous contents, as well as with soil clay, slope, shear and olive age. By contrast, OT, CT and CNT were correlated with mean annual temperature, and the presence of artificial surfaces at landscape level (Fig. 4).

Fig. 4. RDA biplot made with species-abundance data showing the spatial distribution of the plant families (above), and the main environmental variables influencing the accumulated variation (below). In the latter, the treatments are also projected as nominal variables. A = Abandoned, O = Organic, C = Conventional. OT = organic tillage; OC = organic cover crops; CT = conventional tillage; CNT = conventional non-tillage; AC = abandoned cover crops; AW = abandoned woody. CO = soil organic carbon (Mg ha−1), NSOIL = soil organic nitrogen (Mg ha−1), PSOIL = soil phosphorous content (Mg ha−1), PAVG = mean annual precipitation, TAVG = mean annual temperature, SHEAR = soil shear stress (kPa), OLIAGE = olive age, ARTI = artificial surface in the 1 km-radius buffer zone.
Finally, the ordination diagram obtained through the DCA run for the individual abundance of the ‘species × plot’ matrix showed the similarity between treatments according to their species composition and species richness (Fig. 5). In that biplot, treatments including tillage (OT, CT) were poorer in species and had different species composition than non-tilled treatments (OC, CNT). In fact, in terms of species richness and species composition, OC and CNT had more in common with the abandoned olive groves (AW, AC) than with the tilled ones (OT, CT).

Fig. 5. DCA biplot made with species-abundance data showing the spatial ordination of plots according to their similarity in species composition and species richness (indicated by the circle size). Organic tillage (OT) and conventional tillage (CT) are shown with yellow circles, organic cover crop (OC) and conventional non-tillage (CNT) with green circles, and the abandoned treatments (AW, AC) with purple circles.
Discussion
In this study, we evaluate the ground cover and the composition and diversity of the plant species in 20 sloping olive groves (in Andalusia, SE Spain) tended using different farming and soil management systems. These olive groves are subject to a range of different environmental conditions and are at high risk of being abandoned.
Independently of the farming system (organic or conventional) applied in each olive grove, tillage (OT, CT) resulted in a low percentage of vegetation cover, litter and bryophytes on the soil surface. This is because most of these components are either destroyed or buried during tillage. By contrast, very high percentages were recorded in abandoned or untilled olive groves. This contrasts with the results obtained by Maccherini et al. (Reference Maccherini, Santi, Bonini, Amici, Pruscini, Palazzo and Selva2013), who observed an impoverishment of the bryophyte cover in abandoned olive groves with a strong presence of woody species. Vegetation cover assessment shows that OT and CT had soil coverage of less than 30%, the minimum level considered for conservation agriculture systems (Lal, Reference Lal2015). The highest value was obtained by OC with coverage of 73.8%. Meyer et al. (Reference Meyer, Wischmeier and Foster1970) recorded a 1/3 reduction in soil water erosion compared to bare soil when plant debris was used to provide 31% soil cover. Espejo-Pérez et al. (Reference Espejo-Pérez, Rodríguez-Lizana, Ordóñez and Giráldez2013) found practically zero erosion with covers of 70% in olive groves, and Sastre et al. (Reference Sastre, Marques, García-Díaz and Bienes2018) noted a decrease of 80% in soil erosion with Trachynia dystachia coverage of over 80%. Therefore, in the cultivation conditions being studied here, on slopes of 20% or above, which are highly vulnerable to erosion, OC, AC and AW offer very satisfactory levels of soil protection.
In general, the flora of the olive grove is dominated by annual species (Pujadas Salvá, Reference Pujadas Salvá1986; Perrino et al., Reference Perrino, Ladisa and Calabrese2014; Simoes et al., Reference Simoes, Belo, Pinto-Cruz and Pinheiro2014), since one of the main objectives when managing the weedy vegetation that appears in olive groves is to eliminate the perennial species that are considered the greatest competition for the main crop (Pujadas Salvá, Reference Pujadas Salvá1986; Fernández-Quintanilla et al., Reference Fernández-Quintanilla, Mesa García, García-Torres, García-Torres and Fernández-Quintanilla1991). Indeed, the only treatment in which the species with perennial life forms (chamephytes, hemicryptophytes, phanerophytes) were the most abundant was AW, as reported by Fernández-Quintanilla et al. (Reference Fernández-Quintanilla, Mesa García, García-Torres, García-Torres and Fernández-Quintanilla1991) and Simoes et al. (Reference Simoes, Belo, Pinto-Cruz and Pinheiro2014). That means that all the vegetation control operations involving tillage (OT, CT) or herbicide application (CNT) tended to reduce the vascular plant diversity. In short, although from an agronomic point of view, vegetation control has traditionally been considered advisable as a means of increasing olive production (Huqi et al., Reference Huqi, Dhima, Vasilakoglou, Keco and Salaku2009), it has a high environmental cost, especially in sloping agriculture where the topsoil is more vulnerable to erosion.
The dominance of species from the Poaceae, Fabaceae and Asteraceae families was also noted in previous studies of the flora in Mediterranean olive groves (Huqi et al., Reference Huqi, Dhima, Vasilakoglou, Keco and Salaku2009; Solomou and Sfougaris, Reference Solomou and Sfougaris2011; Perrino et al., Reference Perrino, Ladisa and Calabrese2014; Simoes et al., Reference Simoes, Belo, Pinto-Cruz and Pinheiro2014). When vegetation is controlled exclusively by the application of pre-emergence herbicides (CNT), this favored the establishment of annual legumes, such as Medicago minima, M. orbicularis, M. polymorpha, Scorpiurus muricatus and Astragalus hamosus, which germinate well in the fall without the need to bury the seeds (Siles et al., Reference Siles, Torres, Ruiz-Valenzuela and García-Fuentes2016). The widespread presence of annual legumes was reported by Saavedra et al. (Reference Saavedra, Pastor, Arquero and Salas1992) when studying untilled olive groves where the vegetation was controlled with herbicides. The floristic composition of OC (Table 5) presented a balanced composition of Poaceae and Fabaceae, which according to Simoes et al. (Reference Simoes, Belo, Pinto-Cruz and Pinheiro2014) is the ideal cover for soil health and agronomic purposes. This is because the Poaceae species generally produce more biomass and fix more soil carbon due to their fasciculated root system and root renewal rate (Márquez-García et al., Reference Márquez-García, González-Sánchez, Castro-García and Ordoñez-Fernández2013), while the Fabaceae species increase inorganic N in the soil (Rodrigues et al., Reference Rodrigues, Correia, Claro, Ferreira, Barbosa, Moutinho-Pereira and Arrobas2013). A mixture of Poaceae and Fabaceae with a balanced C:N ratio is therefore highly recommended in this sense. In our case, this occurs naturally in the OC treatment, which is also the one with greatest biodiversity.
The species Trachynia distachya was dominant (spontaneously) in AW, OC and AC (Table 6), which explains why so many research studies refer to it and why it has been chosen as a suitable option for those wishing to plant vegetation covers in their olive groves. T. distachya has been used as a cover crop because it germinates in the field without having to be planted under the soil (Jiménez-Alfaro et al., Reference Jiménez-Alfaro, Hernández-González, Fernández-Pascual, Toorop, Frischie and Gálvez-Ramírez2018). It has also been used as a cover crop in olive groves on gypsum soils (Sastre et al., Reference Sastre, Barbero-Sierra, Bienes, Marques and García-Díaz2017, Reference Sastre, Marques, García-Díaz and Bienes2018). Its carbon sequestration capacity in olive grove soils has also been analyzed (Repullo-Ruibérriz de Torres et al., Reference Repullo-Ruibérriz de Torres, Ordóñez, Giráldez, Márquez-García, Laguna and Carbonell-Bojollo2018), and it has been used as a cover crop in conservation agriculture to prevent soil degradation and ensure sustainability (Rodríguez-Lizana et al., Reference Rodríguez-Lizana, Repullo-Ruibérriz de Torres, Carbonell-Bojollo, Alcántara and Ordóñez-Fernández2018).
The dominant species in the tilled olive groves (CT and OT) was Lolium rigidum. Trials with Lolium in which seeds were buried by tillage showed that seeds buried at 10–20 cm have more persistence, deteriorate less and come out before dormancy (Cechin et al., Reference Cechin, Schmitz, Hencks, Monge, Agostinetto and Vargas2021). This explains the dominance of this species and other similar ruderal species in tilled olive groves (Cohen et al., Reference Cohen, Bilodeau, Alexandre, Godron, Andrieu, Grésillon, Garlatti and Morganti2015). In untilled groves, Lolium seeds remain on the surface, where they become a food source for predatory ants (Baraibar et al., Reference Baraibar, Canadell, Torra, Royo-Esnal and Recasens2017). Tillage, however, tends to bury the seeds, which in this way escape their predators. The ants themselves are also affected by tillage, even when tine farrows are used (Campos et al., Reference Campos, Fernández, Ruano, Cotes, Cárdenas and Castro2011; Hevia et al., Reference Hevia, Ortega, Azcárate, López and González2019).
Finally, it is known that frugivorous bird species are responsible for high seed dispersal of fleshy fruit species. It is possible that these birds use olive trees as perches (Rühl et al., Reference Rühl, Caruso, Giucastro and La Mantia2011; González-Varo et al., Reference González-Varo, Carvalho, Arroyo and Jordano2017). That would explain the existence of perennial species with fleshy fruit in the area under the olive tree canopy in AW. Species such as Sylvia atricapilla show a preference for abandoned olive trees rather than those that are intensively cultivated (Assandri et al., Reference Assandri, Morganti, Bogliani and Pulido2017). That could explain why some species were only found in the AW olive groves. Those include Pistacia lentiscus, P. terebintus, Quercus rotundifolia, Rubus ulmifolius, Smilax aspera, Asparagus acutifolius, Rhamnus alaternus and Rubia peregrina among others, characteristic species of the Mediterranean forest and of endozoochorous seed dispersal (Bonet and Pausas, Reference Bonet and Pausas2004). As expected, they are also more abundant in abandoned olive groves because they disappear or are rarer in areas where the vegetation is returned to the early stages of natural succession by soil management (e.g., tillage). In any case the presence of olive trees, which also grow wild in the Mediterranean region, could facilitate the return to the native forest.
In this paper we demonstrate how different farming systems and soil management techniques can affect biological diversity at different levels, in terms of individual abundance, plant cover, species richness and diversity, species and family composition, as well as life forms and species distribution patterns. The soil management systems involving soil tillage (CT, OT) showed the least biodiversity, even in organic olive groves, where the tilled groves (OT) were much poorer in species than those with native plant cover (OC). Similar plant biodiversity results were recorded by Carpio et al. (Reference Carpio, Lora, Martín-Consuegra, Sánchez-Cuesta, Tortosa and Castro2020), albeit only in terms of species richness. However, in a study by Terzi et al. (Reference Terzi, Barca, Cazzato, D'amico, Lasorella and Fracchiolla2021) of olive groves in Apulia (Italy), tillage was not the worst culprit in terms of reducing biodiversity, in that herbicides produced even lower levels, probably due to a more intensive use of herbicides than in our plots (CNT).
In view of our results, there are two possible alternatives to conventional olive cultivation. The first would be the abandonment of marginal sloping olive groves and their conversion into woodland spaces (as has happened in AC and especially AW); and the second would be the conversion to organic farming with native plant cover (OC). The latter could reconcile agricultural activity in sloping olive groves with the conservation of biodiversity and its associated ecosystem services, therefore increasing the sustainability of olive production.
AW and OC were the treatments that showed the greatest coverage and therefore protection of the soil by native vegetation, together with litter and bryophytes. They also favored the coexistence of the greatest abundance of individuals, species and families of vascular plants, and had higher diversity index values and more life forms. The tilled (CT and OT) and conventional non-tilled (CNT) treatments gave rise to unprotected soils and very low biodiversity values, which is quite unsustainable. Despite being organic, OT could be considered ‘non-bioinclusive’, which contradicts the essential principles of organic farming.
In this regard, the combination of farming system and soil management technique in olive groves has rarely been addressed in the literature. Solomou and Sfougaris (Reference Solomou and Sfougaris2011) pointed out that the organic olive grove (grazed and cleared) increases the alpha and beta diversity of herbaceous plants compared to conventional systems (with and without herbicides). In addition, the best chemical and microbiological properties of the soils are obtained in organic olive groves with cover crops of Fabaceae species whose remains are left on the surface (Herencia, Reference Herencia2018). Increases in solitary bees (Martínez-Núñez et al., Reference Martínez-Núñez, Manzaneda, Isla, Tarifa, Calvo, Molina and Rey2019), Lepidoptera Rhopalocera (Sánchez-Fernández et al., Reference Sánchez-Fernández, Vílchez-Vivanco, Navarro and Castro-Rodríguez2020), and birds (Martínez-Núñez et al., Reference Martínez-Núñez, Rey, Manzaneda, Tarifa, Salido, Isla, Pérez, Camacho and Molina2020) have also been reported in organic olive groves with cover crops. In fact, the improved sustainability of organic olive groves with plant cover and mechanical mowing as compared to those with conventional tillage is widely recognized, bringing a range of benefits such as reduced soil erosion and soil organic carbon depletion (Soriano et al., Reference Soriano, Álvarez, Landa and Gómez2014). In this way, organic olive groves with cover crops could provide a solution for enhancing ecosystem services (Keesstra et al., Reference Keesstra, Nunes, Novara, Finger, Avelar, Kalantari and Cerdà2018).
However, the abandonment of sloping olive groves could have a number of disadvantages, such as the loss of direct economic income, which might encourage people to leave rural areas, the loss of the associated sociocultural heritage and the increased risk of fires (Allen et al., Reference Allen, Randall, Amable and Devereux2006). At the same time, it could also be seen as a great opportunity to regenerate patches of forest inside agricultural landscapes, so creating islands of biodiversity of enormous interest as green infrastructure that provides ecosystem services to nearby crops (i.e., biological control of pests), wildlife refuges for endemic or local species and ecological corridors (Paredes et al., Reference Paredes, Cayuela and Campos2013). In fact, Guzmán-Álvarez and Navarro (Reference Guzmán-Álvarez and Navarro-Cerrillo2008) estimated that 75% of marginal olive groves in Andalusia could be afforested solely by natural processes. Our results show that: (i) the abandonment of sloping olive groves would be beneficial from a biological and environmental point of view (unlike other studies which suggested otherwise) and (ii) that in certain landscape contexts it might be advisable to offer farmers financial incentives to encourage them to do so.
For its part, the conversion to organic agriculture with spontaneous or native plant cover could be a very interesting solution to reconcile socioeconomic activity with environmental and biodiversity protection. This could increase the added value of the products and make these marginal olive groves more viable. This would reduce the risk of abandonment, so maintaining the sociocultural and economic benefits for rural areas. It could also improve biodiversity and ecosystem services. Likewise, if farmers were ‘remunerated or encouraged’ to adopt certain soil management techniques that entail improvements in biodiversity, erosion control or carbon sequestration (e.g., native cover crops), that could help prevent the loss of the sociocultural and economic benefits produced by sloping olive groves (CAPDR, 2018; ESYRCE, 2021).
The introduction of plant cover is currently prioritized and subsidized by governments, as is the conversion to organic agriculture, and this could be a great opportunity to monetize sloping olive groves. In this case, plant cover should be mandatory for olive groves (and other woody crops) on slopes of over 20% or perhaps even less, regardless of the farming system. In organic woody crops, tillage should not be allowed, especially on slopes (at present it is permitted under European organic farming regulations, despite being considered harmful for the soil). To this end, we suggest that organic certification schemes should incorporate additional or more specific criteria on farm soil and vegetation management, as proposed by De Leijster et al. (Reference De Leijster, Verburg, Santos, Wassen, Martínez-Mena, de Vente and Verweij2020). The higher prices of organic olive oil (and other organic products such as almonds), together with EU subsidies for organic agriculture, should also help make these sloping olive groves more economically viable.
Finally, the richness, composition and abundance of species and plant families were shown to be influenced by local abiotic characteristics such as mean annual precipitation and temperature, and by certain physical-chemical properties of the soil and landscape complexity, as was quantified by Allen et al. (Reference Allen, Randall, Amable and Devereux2006) in olive groves in Crete (Greece). These authors found that elevation (related to temperature (−) and precipitation (+)), slope, soil pH, organic matter and soil N and P contents were good predictors of plant data variability. For their part, Baessler and Klotz (Reference Baessler and Klotz2006) established a clear relationship between landscape complexity and plant diversity, which is in line with our results in the sense that more homogeneous, more intensively-farmed landscapes (abundance of woody crops, rainfed cereal or artificial surfaces) had a negative correlation with plant diversity. That is probably because simplified landscapes of this kind have less plant diversity, fewer seed sources and more barriers to dispersal. In turn, the environment can be improved by the richness and abundance of the species themselves, which can lead to higher concentrations of soil organic carbon, nitrogen, phosphorous, cation exchange capacity, etc. These aspects should be taken into account in the design of incentive policies aimed at encouraging farmers to convert to certain specific land uses, soil management or farming systems.
Conclusions
In this paper, we have shown that, for biological conservation in sloping olive groves, the soil management techniques used by farmers are more important than the farming system, a finding that partially contradicts hypothesis 1. Specifically, on both conventional and organic farms, treatments involving tillage should not be considered as bio-inclusive or environmentally sustainable. By contrast, treatments with native cover crops had a significant, positive influence on plant diversity, so confirming hypothesis 2. According to this hypothesis, plant diversity was also affected by the environment, especially by climate, soil features and landscape complexity. From an environmental perspective and in the face of increasing competition from intensive olive farming, our recommendation is that the marginal sloping olive groves which are normally managed by tillage should either be abandoned or converted to organic agriculture with spontaneous or native plant cover. The first solution could entail various disadvantages, especially in terms of loss of income, but it could also provide a great opportunity to recover ecosystem services. The second could be a very interesting solution for reconciling socioeconomic activity with environmental and biodiversity protection.
Supplementary material
The supplementary material for this article can be found at https://doi.org/10.1017/S1742170523000091.
Acknowledgements
This study was funded through the INIA project, ‘Sloping olive groves and disadvantaged areas: Environmental and socioeconomic assessment to improve productivity and sustainability (SOLEA-Sloping Olea)’. Project RTA2014-028 with funds from INIA and FEDER 2014-2020 ‘Operational Program of Intelligent Growth’. We thank Nigel Walkington for the English review.
Conflict of interest
Authors have no conflict of interest to declare. There are no disputes over the ownership of the data presented in the paper and all contributions have been attributed appropriately, via coauthorship or acknowledgement, as appropriate to the situation.