Introduction
Following the introduction of DR soybean and cotton cultivars, dicamba has become a critically important tool for controlling many dicotyledonous weed species, including perennial species (Shaner Reference Shaner2014) and glyphosate-resistant (GR) biotypes of Palmer amaranth (Amaranthus palmeri S. Watson), horseweed (Erigeron canadensis L.), and waterhemp [Amaranthus tuberculatus (Moq.) J.D. Sauer] (Beckie et al. Reference Beckie, Ashworth and Flower2019; Behrens et al. Reference Behrens, Mutlu, Chakraborty, Dumitru, Jiang, LaVallee, Herman, Clemente and Weeks2007; Schütte et al. Reference Schütte, Eckerstorfer, Rastelli, Reichenbecher, Restrepo-Vassalli, Ruohonen-Lehto, Wuest-Saucy and Mertens2017). Dicamba is a synthetic auxin herbicide that can be applied preplant in no-till systems, postemergence in grass and DR crops. At least 9 million kg of dicamba were applied to US soybean and cotton (Gossypium hirsutum L.) in 2019 prior to seeding or during the cropping season, whereas less than 5 million kg were applied across all other cropping systems and pastures (USGS 2019).
The widespread use of dicamba has been accompanied by concern for OTM (McCown et al. Reference McCown, Barber and Norsworthy2018; Soltani et al. Reference Soltani, Oliveira, Alves, Werle, Norsworthy, Sprague, Young, Reynolds, Brown and Sikkema2020). Dicamba OTM can happen as particle drift when spray droplets are carried away by the wind from the application area to adjacent fields, as vapor drift when herbicides with high vapor pressure can volatilize, or under atmospheric conditions inducive of air-temperature inversion. In a survey of Nebraska soybean growers, volatility drift was the leading cause of dicamba injury in neighboring non-DR soybean fields (69%), followed by particle drift (23%), and by temperature inversion (8%) (Werle et al. Reference Werle, Oliveira, Jhala, Proctor, Rees and Klein2018). Sensitive broadleaf crops exposed to low rates of dicamba display a distinctive cupping deformation and downward bending (epinasty) of the leaves, swollen petiole bases, and terminal leaf chlorosis, which may result in yield loss (Colquhoun et al. Reference Colquhoun, Heider and Rittmeyer2014; Culpepper et al. Reference Culpepper, Sosnoskie, Shugart, Leifheit, Curry and Gray2018; Griffin et al. Reference Griffin, Bauerle, Stephenson, Miller and Boudreaux2013; Hand et al. Reference Hand, Vance, Randell, Shugart, Gray, Luo and Culpepper2021; McCown et al. Reference McCown, Barber and Norsworthy2018). In 2017, over 1.5 million ha of non-DR soybean in the United States were estimated to have been injured by dicamba exposure (WSSA 2018). Although most reported instances of off-target movement have occurred in the Midwest, the continuous evolution of herbicide-resistant weeds (Heap and Duke Reference Heap and Duke2018) and the associated need for planting DR soybean may result in more frequent cases of crop injury in the Mid-Atlantic region. For example, in response to GR weeds such as Palmer amaranth (Amaranthus palmeri S. Watson) and common ragweed (Ambrosia artemisiifolia L.), DR soybean acreage has increased in New Jersey. In 2021, approximately 70% of the 38,000 total soybean hectares were planted to DR cultivars (E. Guenther, Nutrien Ag Solutions, personal communication).
In 2020, more than 810,000 ha of vegetables were grown in the United States with a farm-gate value of over $13 billion (USDA-NASS 2020). The agricultural census estimated that New York, New Jersey, and Delaware had $378 million, $222 million, and $60 million in market sales of vegetable crops in 2017, respectively (USDA-NASS 2017). Fruits and vegetables in the Mid-Atlantic regions are often grown close to DR soybean fields, increasing the risk of dicamba exposure onto vulnerable crops. Previous literature has shown that Fabaceae, Solanaceae, and Cucurbitaceae crops are sensitive, to varying degrees, to dicamba. Hand et al. (Reference Hand, Vance, Randell, Shugart, Gray, Luo and Culpepper2021) reported that dicamba applied at 7.5 g ae ha–1 (1/75 of the soybean maximum use rate) to cucumber at the one- to two-leaf stage reduced vine length by 23% and fruit count by 16% compared to a nontreated control. Dicamba applied at the vegetative growth stage at 2.24 g ae ha–1 (1/250 of the soybean maximum use rate) and 7.5 g ae ha–1 reduced watermelon yield between 13% and 20% (Culpepper et al. Reference Culpepper, Sosnoskie, Shugart, Leifheit, Curry and Gray2018). Knezevic et al. (Reference Knezevic, Osipitan and Scott2018) showed that tomato (Solanum lycopersicum L.) plant height decreased by up to 50% when exposed to 11.2 g ae ha–1 (1/50 of the soybean maximum use rate). Kruger et al. (Reference Kruger, Johnson, Doohan and Weller2012) indicated that early-bloom applications of dicamba at 7.5 g ae ha–1 caused 25% yield reduction in processing tomatoes. Dicamba at 1.4 (1/400 of soybean maximum use rate) to 11.2 g ae ha–1 delayed bell pepper maturity, with fruit yield decreasing 59% to 82% at the first harvest, but did not affect the cumulated yield (Mohseni-Moghadam and Doohan Reference Mohseni-Moghadam and Doohan2015). If previous studies have investigated the effects of simulated dicamba drift rate on pepper and tomatoes (Baurle et al. Reference Baurle, Griffin, Alford, Curry and Kenty2017; Dittmar et al. Reference Dittmar, Ferrell, Fernandez and Smith2016; Knezevic et al., Reference Knezevic, Osipitan and Scott2018; Kruger et al. Reference Kruger, Johnson, Doohan and Weller2012; Mohseni-Moghadam and Doohan Reference Mohseni-Moghadam and Doohan2015; Warmund et al. Reference Warmund, Ellersieck and Smeda2022), no prior research work has been conducted on eggplant. For leguminous vegetable crops, Colquhoun et al. (Reference Colquhoun, Heider and Rittmeyer2014) reported that dicamba at 1.4 g ae ha–1 on snap bean caused 43% injury 4 WAT and 81% total yield reduction.
A previous greenhouse study evaluated 12 different vegetable crops in the Cucurbitaceae, Solanaceae, and Fabaceae families for sensitivity to low doses of dicamba (Wasacz et al. Reference Wasacz, Ward, VanGessel and Besançon2022). Because crop sensitivity to dicamba may differ depending on environmental conditions, the three most sensitive crop species in each botanical family identified in this greenhouse study were selected for further field evaluation at three different locations representative of agronomic and meteorological conditions of the northeastern United States. As fruit production was not documented in the greenhouse study, the objective of the field study was to (1) visually assess injury; (2) measure plant development or biomass production; and (3) determine the quantitative and qualitative effect on yield in response to simulated drift rates of dicamba applied at two separate growth stages of cucumber, eggplant, and snap bean.
Materials and Methods
Experiments to describe the effects of dicamba application timing and simulated drift rates on crop injury, growth, and yield were conducted in 2020 and 2021 at the Rutgers Agricultural Research and Extension Center near Bridgeton, NJ (39.53°N, 75.20°W), the New York Agricultural Experiment Station in Geneva, NY (42.88°N, 77.01°W), and the University of Delaware Carvel Research and Education Center near Georgetown, DE (38.64°N, 75.46°W). Local soils were a Chillum silt loam (fine-silty, mixed, semiactive, mesic Typic Hapludults) with 13% sand, 69% silt, 18% clay, 1.7% organic matter, and a pH of 5.0 at Bridgeton, a Honeoye loam (fine-loamy, mixed, semiactive, mesic Glossic Hapludalfs) with 38% sand, 44% silt, 18% clay, 2.5% organic matter, and a pH of 6.3 at Geneva, and a Rosedale loamy sand (loamy, siliceous, semiactive, mesic Arenic Hapludults) with 81% sand, 14% silt, 5% clay, 1% organic matter, and a pH of 5.8 at Georgetown.
Cucumber ‘Python’ (Seedway, LLC, Hall, NY) was single-row seeded at an average depth of 2.5 cm with an in-row spacing of 30 cm into previously disked flat beds on July 6, 2020, and June 17, 2021, near Bridgeton, and on May 24, 2021, near Georgetown. Cucumber trials were treated with a premix (Strategy®; Loveland Products, Inc., Greeley, CO) of clomazone (0.21 kg ai ha–1) and ethalfluralin (0.67 kg ai ha–1) applied immediately after cucumber seeding at a spray volume of 140 L ha–1.
Eggplant ‘Santana’ (Seedway, LLC, Hall, NY) was single-row transplanted at the one- to two-leaf stage into raised beds with an in-row spacing of 61 cm on June 6, 2020, and June 8, 2021, near Bridgeton. Root balls were set at a depth of 5 cm. Eggplant trials received 2.24 kg ai ha–1 of napropamide (Devrinol 2-XT®; United Phosphorus, King of Prussia, PA) applied at a spray volume of 140 L ha–1 72 h prior to transplanting. Cucumber and eggplant were planted on bare ground rather than using plastic mulch to prevent dicamba from contacting the plastic and potentially volatilizing.
Snap bean ‘Caprice’ (Seedway, LLC, Hall, NY) was single-row seeded on flat beds on June 29, 2020, and July 9, 2021, near Bridgeton. The average seeding depth and in-row spacing were 2.5 cm and 5 cm, respectively. Snap bean ‘Huntington’ (Syngenta Seeds, Greensboro, NC) was seeded on flat beds in double rows spaced 76 cm apart on June 26, 2020, and June 25, 2021, near Geneva. The average seeding depth and in-row spacing were 3.8 cm and 5 cm, respectively. Both snap bean trials received 2.14 kg ai ha–1 of S-metolachlor (Dual Magnum®; Syngenta Crop Protection, Greensboro, NC) immediately after seeding. The ‘Caprice’ beans also received 53 g ai ha–1 of halosulfuron (Sandea®; Gowan Company, Yuma, AZ) in combination with S-metolachlor. Residual herbicides were incorporated with 0.9 to 1.7 cm overhead irrigation or rainfall within 96 h of application.
Individual plot size was 1.5 by 6 m for all crop species. Plots were hand-weeded or cultivated as necessary to prevent weed competition. Management of insects and disease was conducted in accordance with university recommendations for the Mid-Atlantic region (Wyenandt et al. Reference Wyenandt, Owens, Johnson, Hamilton, Hastings and VanGessel2022). Trials were fertilized prior to planting with 60 kg N ha–1.
All trials were conducted as a two-factor factorial arrangement in a randomized complete block design with four replications. Main factors were dicamba application timing (vegetative or early reproductive growth stage) and rate (0.056 to 2.24 g ae ha–1). Applications were made over the top of each crop either at the second to third true-leaf (V2) or the early reproductive (R1) stages. Application dates are indicated in Table 1. Dicamba (XtendiMax®; Bayer Crop Science, St. Louis, MO) was applied at 0.056, 0.11, 0.56, 1.12, 2.24 g ae ha–1, respectively, corresponding to 1/10,000, 1/5,000, 1/1,000, 1/500, and 1/250 of the maximum recommended label rate (560 g ae ha–1) for soybean. These rates were selected based on the lowest rate of dicamba (0.056 g ae ha–1) that induced a phytotoxicity response in sensitive soybean plants (Egan and Mortensen Reference Egan and Mortensen2012) as well as rates previously evaluated in a greenhouse study with these vegetable species (Wasacz et al. Reference Wasacz, Ward, VanGessel and Besançon2022). Over-the-top applications of dicamba were made using CO2 -pressurized backpack sprayers calibrated to deliver 140 L ha–1 at 179 kPa for eggplant, and 187 L ha–1 at 138 kPa for cucumber and snap bean. Eggplant was sprayed using 11002 AIXR nozzles (TeeJet Technologies, Wheaton, IL), whereas cucumber and snap bean were treated using 11003 TTI nozzles (TeeJet Technologies, Wheaton, IL). Booms were 138 cm long with nozzles spaced 46 cm apart and a boom height of 46 cm above the crop canopy. All experiments also included a nontreated control for comparison purpose. Plots were separated in space by guard rows or 1.5-m-wide alleys. Air temperature and relative humidity at the time of application ranged from 25 C to 32 C and from 42% to 76%, respectively, and wind speed did not exceed 4.8 km h–1.
Table 1. Dicamba application dates at the vegetative (V2) and reproductive (R1) stages for eggplant, cucumber, and snap bean in Bridgeton, NJ, Geneva, NY, and Georgetown, DE, in 2020 and 2021.

a Abbrevations: DE, Delaware; NJ, New Jersey; NY, New York.
Crop injury, a composite estimation of leaf deformation, stunting, and epinasty, was visually assessed on a scale from 0 to 100%, with 0% being no visible injury and 100% being total plant death. Visible injury in cucumber, eggplant, and ‘Caprice’ snap bean was evaluated 2 and 4 WAT following each application. ‘Huntington’ snap bean injury was evaluated 2 WAT following each application. At Bridgeton, cucumber vine length (5 plants per plot) was measured from the base of the plant to the tip of the vine to assess crop stunting 4 WAT. Snap bean height was measured 2 WAT in Bridgeton (10 plants per plot) both years, and 10 d after each application timing in Geneva (20 plants per plot). Cucumber and eggplant yield data were collected from 5 center plants per plot. Plants were harvested once a week for 5 consecutive wk. Individual fruits were counted, weighed, and categorized into No. 1, No. 2, and cull-size classes (USDA-AMS 2013, 2018). ‘Caprice’ snap bean was harvested from a 3.7-m length of row in the center of each plot on August 31, 2020, and September 10, 2021; these timings occurred beyond optimal marketing quality. ‘Huntington’ snap bean was harvested at optimal commercial quality from the center 1.5 m of each row in each plot on August 13, 2020, and August 17, 2021. Snap bean pods at both locations were separated into marketable and cull categories (USDA-AMS 1997) and weighed. The number of pods per plant was also counted for ‘Caprice’ snap bean. Snap bean plant biomass was collected at harvest by clipping plants at the soil level, and fresh weights were recorded. Individual plant weights were used as an additional assessment of snap bean growth response to dicamba. Within each crop species and dicamba timing of application, relative commercial yield (RCY) was computed for each dicamba rate following Equation 1:

Where CY i,t is the commercial yield for dicamba rate I and block n, and CY 0,n is the commercial yield for the nontreated control and block n.
Averages of subsamples collected from each individual plot was used for analysis of plant height or length. Due to unequal variances, crop injury data were arcsine square root transformed prior to analysis and back-transformed for data presentation (Grafen and Hails Reference Grafen and Hails2002). Data were subjected to ANOVA using PROC GLIMMIX in SAS version 9.4 (SAS Institute, Cary, NC) to evaluate the effects of dicamba rate, application timing, and their interaction on visual estimates of injury, plant height, vine length, vegetative biomass, and crop yield. Year and location were combined into environments and considered to be randomly sampled from a population (Carmer et al. Reference Carmer, Nyquist and Walker1989). As suggested by Blouin et al (Reference Blouin, Webster and Bond2011), environment, replications, and replications nested within environment were considered random effects. Dicamba rate, application timing, snap bean cultivars, and their interactions were considered fixed effects. In the absence of significant interactions, data were combined over fixed effects. Mean comparisons between treatments were performed using Fisher’s protected LSD at α = 0.05).
Results and Discussion
Because of cultivar-by-rate and cultivar-by-rate-by-application timing interactions for the different ratings, snap bean data were analyzed by cultivar (Table 2). Injury for cucumber and eggplant, cucumber vine length, snap bean heights, snap bean biomass, and crop yields were affected by dicamba rate. Developmental stage at the time of application influenced cucumber vine length, snap bean height, and ‘Huntington’ commercial yield. Dicamba rate-by-crop stage interaction was significant for injury 2 WAT for both snap bean cultivars and 4WAT for ‘Caprice’ snap bean.
Table 2. ANOVA for injury, plant growth, biomass, and yield for cucumber, eggplant, and snap bean. Data were combined from trials conducted in 2020 and 2021 at Bridgeton, NJ, Georgetown, DE, and Geneva, NY. Dicamba at 0.056, 0.11, 0.56, 1.12, and 2.24 g ae ha–1 was applied at V2 and R1 growth stages to each vegetable crop.

a Bold type indicates P values significant at P < 0.05.
b Abbreviations: plt, plant; RCY, relative commercial yield; TFB, total fresh biomass; WAT, weeks after treatment.
Cucumber
Cucumber showed minimal crop injury (1% to 3%) 2 and 4 WAT, but only at rates of 1.12 g ae ha–1 and higher (Table 3). Averaged over application timings and compared to the nontreated control, dicamba rate equal or higher than 1.12 g ae ha–1 reduced cucumber vine length 2 WAT and commercial yield by 9%, on average. Lower dicamba rates had no effect on plant growth or commercial yield. Vine length was affected by application timing with 38% longer vine at R1 than at V2 stage; this is because dicamba applications were staggered over time and R1 plants were larger than V2 plants at treatment (data not shown).
Table 3. Main effect of dicamba rate on injury, plant growth, and yield for ‘Python’ cucumber. Data are combined over application timings (V2 and R1) for trials conducted in 2020 and 2021 at Bridgeton, NJ, and in 2021 at Georgetown, DE.

a Injury rated on a scale from 0% to 100%, with 100% corresponding to plant death.
b Abbreviations: RCY, relative commercial yield; WAT, weeks after treatment.
c Means followed by the same letter within a column are not significantly different based on Fisher’s protected LSD (α = 0.05).
d Asterisk indicates means significantly different from the nontreated control. The nontreated control averaged 88 cm vine length and 1,430 g plant–1 commercial yield.
Early-season cucumber production (first and second harvests) was evaluated to determine if dicamba could delay maturity as has been observed in watermelon (Culpepper et al. Reference Culpepper, Sosnoskie, Shugart, Leifheit, Curry and Gray2018), cotton (Byrd et al. Reference Byrd, Collins, Culpepper, Dodds, Edmisten, Wright, Morgan, Baumann, Dotray and Manuchehri2016), and soybean (Scholtes et al. Reference Scholtes, Sperry, Reynolds, Irby, Eubank, Barber and Dodds2019). Early-season marketable yield and total fruit count were not affected by dicamba rate. However, when averaged across dicamba rates, application at V2 reduced early-season marketable yield and total fruit count by 17% and 12%, respectively, compared to application made at R1 (data not shown).
Previous studies reported greater cucumber yield reductions by dicamba than noted in these trials. For example, Hand et al. (Reference Hand, Vance, Randell, Shugart, Gray, Luo and Culpepper2021) showed that dicamba applied at 7.5 g ae ha–1 to ‘Bristol’ and ‘Impact’ cultivars reduced average fruit number and vine length by 48% and 23%, respectively. However, growth or yield were not reduced at the 2.24 g ae ha–1 rate. In a greenhouse screening conducted prior to this study, Wasacz et al. (Reference Wasacz, Ward, VanGessel and Besançon2022) determined that ‘Python’ was less sensitive to low rates of dicamba than ‘Burpless Beauty’ cultivar, requiring 1.12 g ae ha–1 and 0.28 g ae ha–1, respectively, to induce greater leaf deformation than noted for the nontreated control. It is not known why differences in dicamba sensitivity were observed in ‘Python’ cucumber between the greenhouse and field trials, although abiotic conditions, such as temperature, wind, or humidity, could have influenced the interaction between crop and dicamba.
Eggplant
Only the main effect of dicamba rate was significant for eggplant injury 2 and 4 WAT and the total number of fruits per plant, whereas both dicamba rate and crop stage were significant for RCY (Tables 2 and 4). Averaged across application timings, higher crop injury 2 WAT was observed with dicamba at 1.12 g ae ha–1 (22%) and 2.24 g ae ha–1 (35%) compared to lower rates for which crop injury did not exceed 9% (Table 4). At 4 WAT, injury of 5%, 8%, and 14% was observed where dicamba was applied at 0.56, 1.12 and 2.24 g ae ha–1, respectively.
Table 4. Main effect of dicamba rate and crop stage on injury and yield for ‘Santana’ eggplant. Data are combined over years for trials conducted in 2020 and 2021 at Bridgeton, NJ.

a Injury rated on a scale from 0% to 100%, with 100% corresponding to plant death.
b Abbreviations: plt, plant; R1, early reproductive stage; RCY, relative commercial yield; V2, early vegetative stage; WAT, weeks after treatment.
c Means followed by the same letter within a column are not significantly different based on Fisher’s protected LSD (α = 0.05).
d Asterisk indicates means significantly different from the nontreated control. The nontreated control averaged 2,030 g plant–1 commercial yield and 3.9 fruits per plant.
Averaged across application timings, only dicamba at 2.24 g ae ha–1 reduced RCY (27%) and fruit numbers (31%) as compared to the nontreated control. Averaged across rates, eggplant was more sensitive to dicamba applied at R1 than at V2 with RCY reduced by 19%. Early-season (first and second harvest) marketable yield and total fruit count were not affected by dicamba rates or by application timings (data not shown).
Previous studies focusing on solanaceous crops have shown a wide range of yield responses to simulated drift rates of dicamba. Reminiscent of what was observed for eggplant in this study, Kruger et al. (Reference Kruger, Johnson, Doohan and Weller2012) reported greater tomato sensitivity to dicamba at early bloom compared to the vegetative stage, with 25% yield reduction occurring at 7.5 g ae ha–1 and 11.9 g ae ha–1, respectively. Mohseni-Moghadam and Doohan (Reference Mohseni-Moghadam and Doohan2015) did not observe a reduction of total yield following exposure of bell pepper (Capsicum annuum L.) at the 10-leaf stage to dicamba rates ranging from 1.4 to 11.2 g ae ha–1. However, yield at first harvest was reduced up to 74%, regardless of rate, suggesting that sublethal rates of dicamba could delay fruit ripening.
The present study demonstrates that substantial yield reduction can occur when eggplant is exposed to dicamba at 2.24 g ae ha–1 or at the reproductive stage, suggesting a low tolerance of eggplant to dicamba drift. This corroborates the results of a previous greenhouse study that reported lower dicamba tolerance of ‘Santana’ eggplant compared to ‘Roma’ tomato or ‘Great Stuff’ bell pepper. Each of these solanaceous crops required dicamba doses of 0.11, 0.28, and 0.56 g ae ha–1, respectively, to induce leaf deformation greater than noted for the nontreated control (Wasacz et al., Reference Wasacz, Ward, VanGessel and Besançon2022).
‘Caprice’ Snap Bean
The rate-by-application timing interaction was significant for injury 2 WAT (P < 0.0001) and 4 WAT (P = 0.0476). In this study, ‘Caprice’ snap bean showed considerable symptomatic response to dicamba exposure occurring at the V2 stage with injury 2 WAT ranging from 5% at 0.11 g ae ha–1 to 45% at 2.24 g ae ha–1 (Table 5). Similar levels of snap bean injury (38% to 53%) were reported 2 WAT by Colquhoun et al. (Reference Colquhoun, Heider and Rittmeyer2014) following dicamba rates ≥1.14 g ae ha–1 applied at the one- to two-trifoliate leaf growth stage. Less injury was noted following the R1 application timing with maximum injury 2 WAT of 8% at the 2.24 g ae ha–1 rate. By 4 WAT, injury was 7% on average following the V2 application timing for dicamba at 0.56 g ae ha–1 or greater (Table 5). In contrast, injury increased between 2 and 4 WAT for snap bean treated at the R1 stage with dicamba ≥0.56 g ae ha−1 (10% to 28%). We hypothesize that the delayed onset of dicamba injury following R1 application compared to V2 could be caused by greater investment of crop nutritional resources into production of reproductive than vegetative structures at R1 compared to V2 stage. Because fewer new leaves are produced at R1 than at V2 stage, it may take more time for dicamba-induced injury to show up.
Table 5. Interaction effect of dicamba rate and crop stage on injury for ‘Caprice’ and ‘Huntington’ snap bean a . Data are combined over years for trials conducted in 2020 and 2021 at Bridgeton, NJ, and at Geneva, NY.
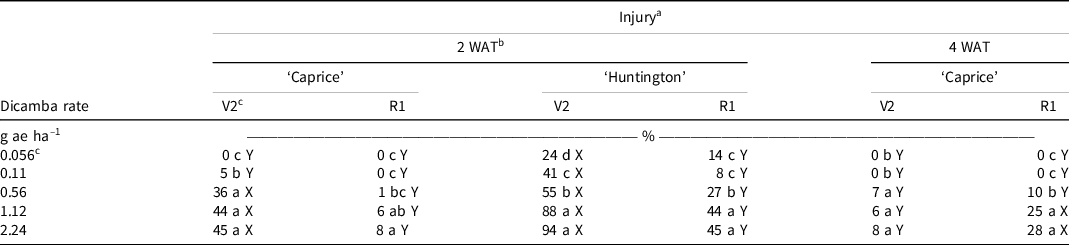
a Injury rated on a scale from 0% to 100%, with 100% corresponding to plant death.
b Abbreviations: R1, early reproductive stage; V2, early vegetative stage; WAT, weeks after treatment.
c Means followed by the same letter in a column (a–c) or row within cultivars (X–Y) are not significantly different based on based on Fisher’s protected LSD (α = 0.05).
‘Caprice’ snap bean height 2 WAT was reduced 19% with dicamba at 1.12 or 2.24 g ae ha–1 compared to the nontreated control or rates ≤0.11 g ae ha–1 (Table 6). Greater plant height was observed 2 WAT following applications at R1 compared to V2. Dicamba rates ≥0.56 g ae ha–1 reduced plant biomass at harvest by 24% on average compared to the nontreated control and across crop stage at the time of application (Table 6).
Table 6. Main effect of dicamba rate and crop stage on plant height, biomass, and yield for ‘Caprice’ snap bean. Data are combined over years for trials conducted in 2020 and 2021 at Bridgeton, NJ.

a Abbreviations: plt, plant; R1, early reproductive stage; RCY, relative commercial yield; TFB, total fresh biomass; V2, early vegetative stage; WAT, weeks after treatment.
b Means followed by the same letter within a column are not significantly different based on Fisher’s protected LSD (α = 0.05).
c Asterisk indicates means significantly different from the nontreated control. The nontreated control averaged 30 cm height, 270 g plant–1 total fresh biomass, 130 g plant–1 commercial yield, 26 beans per plant, and 7% culls.
RCY was also affected by dicamba rate. Averaged across application timings, 30% commercial yield loss occurred following dicamba applied at rates ≥1.12 g ae ha–1. This yield loss was associated with a greater proportion (8%) of nonmarketable beans and a total number of beans produced per plant reduced by 17% compared to the nontreated control (Table 6).
‘Huntington’ Snap Bean
Like ‘Caprice’ snap bean, the rate-by-application timing interaction was significant for injury 2 WAT (P = 0.0286). For the V2 application, injury ratings ranged from 25% at a dicamba rate of 0.056 g ae ha–1 to 94% at 2.24 g ae ha–1 (Table 5). Applications of dicamba at the R1 stage resulted in less injury, compared to the V2 timing, although mean injury of 44% and 45% was observed at rates of 1.12 and 2.24 g ae ha–1 (Table 5). ‘Huntington’ bean results are consistent with the ‘Caprice’ snap bean experiment as well as Griffin et al. (Reference Griffin, Bauerle, Stephenson, Miller and Boudreaux2013), who found that soybean treated with low rates of dicamba resulted in higher injury levels at the vegetative timing application compared to the reproductive timing application. Height reductions at 2 WAT translated into plant biomass reductions at harvest (Table 7). Averaged over timings, less biomass was recorded for plants treated with dicamba at rates of 0.56, 1.12, and 2.24 g ae ha–1.
Table 7. Main effect of dicamba rate and crop stage on plant height, biomass, and yield for ‘Huntington’ snap bean. Data are combined over years for trials conducted in 2020 and 2021 at Geneva, NY.

a Abbreviations: plt, plant; R1, early reproductive stage; RCY, relative commercial yield; TFB, total fresh biomass; V2, early vegetative stage; WAT, weeks after treatment.
b Means followed by the same letter within a column are not significantly different based on Fisher’s protected LSD (α = 0.05).
c Asterisk indicates means significantly different from the nontreated control. The nontreated control averaged 47 cm height, 120 g plant–1 total biomass production, 60 g plant–1 commercial yield, and 11% culls.
RCY was similarly affected; the amounts of marketable beans decreased with increasing dicamba rates >0.056 g ae ha–1. Correspondingly, the amounts of cull beans increased with increasing rate. Observations of yield loss are in line with estimates from Colquhoun et al. (Reference Colquhoun, Heider and Rittmeyer2014), who reported ‘Hercules’ snap bean yield was reduced by 83% with dicamba applied at 1.4 g ae ha–1 at the one- to two-trifoliate stage. For ‘Huntington’ bean, R1 applications of dicamba resulted in greater marketable yield loss compared to V2 treatments (Table 7). This agrees with a 2014 study indicating that soybean was more sensitive to yield reduction when dicamba was applied at the reproductive stage compared to the vegetative stage (Solomon and Bradley, Reference Solomon and Bradley2014). The variability among studies with respect to injury and yield loss in leguminous crops is likely due to differences in production environments but may also be a function of species and cultivar sensitivity (Colquhoun et al. Reference Colquhoun, Heider and Rittmeyer2014; Griffin et al. Reference Griffin, Bauerle, Stephenson, Miller and Boudreaux2013; Kniss Reference Kniss2018; Solomon and Bradley et al. Reference Solomon and Bradley2014). For example, Wasacz et al. (Reference Wasacz, Ward, VanGessel and Besançon2022) reported that ‘Bush Blue Lake 274’ snap bean was less sensitive to dicamba compared to ‘Caprice’, with 0.28 g ae ha–1 and 0.11 g ae ha–1, respectively, required to induce greater leaf deformation than noted for the nontreated control.
In conclusion, off-target movement of dicamba to snap bean poses the greatest risk for injury, plant growth and biomass accumulation, and yield loss. Both snap bean cultivars experienced severe yield loss at rates as low as 0.56 and 1.12 g ae ha–1. Whereas McCown et al. (Reference McCown, Barber and Norsworthy2018) and Meyeres et al. (Reference Meyeres, Lancaster, Kumar, Roozeboom and Peterson2021) reported soybean yield loss of 7% to 34% for dicamba at 2.18 to 8.75 g ae ha–1, our results indicate that snap bean may be more sensitive to dicamba than soybean with up 93% yield loss at 2.24 g ae ha–1. These field data confirm observations from a previous greenhouse study (Wasacz et al. Reference Wasacz, Ward, VanGessel and Besançon2022) and support the need for additional dicamba application restrictions when snap bean is planted nearby DR soybean. Conversely, ‘Python’ cucumber demonstrated good tolerance within the range of dicamba rates tested in this study, with minimal to no injury and yield loss not exceeding 12% in agreement with previous reports (Wasacz et al. Reference Wasacz, Ward, VanGessel and Besançon2022). However, this does not preclude that greater yield reduction may occur at higher rates, as noted by Hand et al. (Reference Hand, Vance, Randell, Shugart, Gray, Luo and Culpepper2021) with dicamba applied at 7.5 g ae ha–1. Previous studies conducted on soybean have shown that dicamba sensitivity can vary between cultivars (Weidenhamer et al. Reference Weidenhamer, Triplett and Sobotka1989, McCown et al. Reference McCown, Barber and Norsworthy2018, Meyeres et al. Reference Meyeres, Lancaster, Kumar, Roozeboom and Peterson2021). We hypothesize that a similar interaction may occur for cucumber, warranting the need for further evaluation of dicamba response across multiple cucumber cultivars. Eggplant showed considerable injury response to dicamba rate as low as 1.12 g ae ha–1. However, yield loss only occurred at the highest rate of 2.24 g ae ha–1, suggesting that injury may not be indicative of potential eggplant yield reduction.
Acknowledgments
The authors acknowledge funding support for this research by Bayer Crop Science, the Rutgers New Jersey Agricultural Experiment Station, and the New York Vegetable Research Association. The authors would also like to express their appreciation for technical support provided by Jim Cicalese (Seedway, LLC), Baylee Carr, Tim Jensen, Suzanne Polashock, Don’tea Robinson, Winston Teo, David Mayonado, Corrie Hopkins, and the staff of the Rutgers Agricultural Research and Extension Center. The authors would also like to acknowledge the efforts of Elizabeth Maloney, Melissa McClements, Sophia Shaw, Liam Murphy, Matthew Christensen, and the staff of the Field Research Unit at Cornell AgriTech. No conflicts of interests have been declared.