Introduction
Mexico has ~1,567,300 ha of coastal wetlands, of which about 674,500 ha (43%) lie along the Gulf of Mexico (GoM) (including the Atlantic Ocean and the Caribbean Sea) (Contreras-Espinosa & Warner, Reference Contreras-Espinosa and Warner2004). These are represented by about 149 geomorphological forms, of which 59% are coastal lagoons (De La Lanza Espino et al., Reference De La Lanza Espino, Pérez and Pérez2013). Coastal lagoons are shallow, enclosed or semi-enclosed coastal wetlands, which are ephemeral or permanently flooded, and usually separated from the sea by a sand barrier (Lankford, Reference Lankford1977; Kjerfve, Reference Kjerfve1994). Coastal lagoons are often characterized by strong spatial salinity gradients (Lamptey & Armah, Reference Lamptey and Armah2008) and temporal variations in salinity at different time scales, the latter related to intra-annual fluctuations in the precipitation-evaporation balance, tidal forces and wind stress, all of which regulate the hydrological balance (Kjerfve & Magill, Reference Kjerfve and Magill1989). Such lagoons are often highly productive aquatic systems that contain many habitat types (Herrera-Silveira & Morales-Ojeda, Reference Herrera-Silveira and Morales-Ojeda2010) and therefore support numerous invertebrate phyla (Hernández-Guevara et al., Reference Hernández-Guevara, Pech and Ardisson2008).
Molluscs are an abundant and diverse phylum in coastal lagoons because the numerous taxa collectively display a broad range of optima with respect to ambient conditions, e.g. salinity and temperature, and some individual species display a broad tolerance with respect to those variables (Wingard & Surge, Reference Wingard and Surge2017). Temperature is perhaps the most important environmental variable that determines large-scale geographic distributions of marine organisms, whereas salinity exerts greater control at the local level (Turney & Perkins, Reference Turney and Perkins1972; Lamptey & Armah, Reference Lamptey and Armah2008; Tunnell et al., Reference Tunnell, Andrews, Barrera and Moretzsohn2010; Selly et al., Reference Selly, Hale, Schiffbauer, Clapp and Huntley2018; De Jesús-Carrillo et al., Reference De Jesús-Carrillo, Ocaña, Hernández-Ávila, Mendoza-Carranza, Sánchez and Barba-Macías2020, Reference De Jesús-Carrillo, Ocaña and Barba-Macías2021). In addition to temperature and salinity, other environmental factors affect benthic mollusc distributions, such as water circulation (Tunnell et al., Reference Tunnell, Andrews, Barrera and Moretzsohn2010; Grenz et al., Reference Grenz, Fichez, Silva, Benítez, Conan, Esparza, Denis, Ruiz, Douillet, Martinez, Ghiglione, Mendieta, Origel-Moreno, Garcia, Caravaca, Pujo-Pay, Alvarado and Zavala-Hidalgo2017), substrate type (Abdelhady et al., Reference Abdelhady, Khalil, Ismail, Fan, Zhang and Xiao2019), habitat complexity (De Duarte et al., Reference de Duarte, Mota and Dias2020) and oxygen concentration (De Jesús-Carrillo et al., Reference De Jesús-Carrillo, Ocaña, Hernández-Ávila, Mendoza-Carranza, Sánchez and Barba-Macías2020).
Benthic mollusc taxa occupy many positions in the food web. Most bivalves are suspension feeders, whereas gastropods, which display infaunal and epifaunal benthic life habits, are primarily grazers, predators or scavengers (de Lucena et al., Reference de Lucena, Oliveira de Meirelles and Matthews-Cascon2012; Wingard & Surge, Reference Wingard and Surge2017). Molluscs are also a preferred food source for many other organisms (Arceo-Carranza et al., Reference Arceo-Carranza, Vega-Cendejas and Hernández de Santillana2013; Chi-Espínola & Vega-Cendejas, Reference Chi-Espínola and Vega-Cendejas2013; Hinojosa-Garro et al., Reference Hinojosa-Garro, Arceo-Gómez, Zambrano and Escalera-Vázquez2013).
Several investigations yielded lists of molluscs from the coastal GoM (Vokes & Vokes, Reference Vokes and Vokes1983; García-Cubas & Reguero, Reference García-Cubas and Reguero2004, Reference García-Cubas and Reguero2007). For coastal lagoons, a great deal of information from the western and southern GoM was generated from the 1970s to 2000. It consists of taxonomic inventories, as well as studies on community structure and ecology (García-Cubas, Reference García-Cubas1970, Reference García-Cubas1971, Reference García-Cubas1981; Reguero & García-Cubas, Reference Reguero and García-Cubas1989, Reference Reguero and García-Cubas1991b, Reference Reguero and García-Cubas1993, Reference Reguero and García-Cubas1994; García-Cubas et al., Reference García-Cubas, Escobar de la Llata, Gonzalez Ania and Reguero1990, Reference García-Cubas, Reguero and Elizarrarás1992, Reference García-Cubas, Escobar and Reguero1999). Recently, the assemblage structure and functional ecology of molluscs was addressed in Mecoacn Lagoon, also in the southern GoM (De Jesús-Carrillo et al., Reference De Jesús-Carrillo, Ocaña, Hernández-Ávila, Mendoza-Carranza, Sánchez and Barba-Macías2020, Reference De Jesús-Carrillo, Ocaña and Barba-Macías2021). An exhaustive evaluation of the malacological diversity associated with different habitats was performed in Los Petenes Biosphere Reserve, Campeche (Mexico), and in a suite of coastal lagoons along the western and south-eastern Mexican GoM (Lagunas Mandinga, Sontecomapan, Carmen-Machona, Mecoacán, Tupilco-Ostión, Terminos, Atasta, Santa Anita and Sabancuy) (Gonzalez et al., Reference Gonzalez, Chávez, de la Cruz and Torruco2018). Nevertheless, studies of molluscs in the eastern GoM (i.e. the Yucatán Peninsula and Caribbean coastal wetlands) are few. The geographic distribution patterns and species richness of bivalves and gastropods from 33 localities along the shoreline (including some coastal wetlands) and corals reefs of the Yucatán Peninsula were studied about 30 years ago, using specimens collected during the 1980s (Gonzalez et al., Reference Gonzalez, Chávez, de la Cruz and Torruco1991). In the last decade, a study addressing the entire macrobenthic fauna community in the Río Lagartos coastal lagoon, northern Yucatán Peninsula, yielded information on the presence, patterns of distribution, and physical-chemical controls on the dominant molluscs (Kuk-Dzul et al., Reference Kuk-Dzul, Gold-Bouchot and Ardisson2012). Aside from those studies, however, we lack critical information, such as functional traits, updated inventories, or ecological preferences, about molluscs in coastal lagoons of the eastern GoM (Yucatán Peninsula).
The coastal zone of the Yucatán Peninsula is often recognized as a characteristic unit, with two subregions that have very different oceanographic characteristics: (i) the northern and western parts of the Yucatán Peninsula, which are part of the Gulf of Mexico, and (ii) the eastern coast of the Peninsula, which is part of the Caribbean Sea (Herrera-Silveira et al., Reference Herrera-Silveira, Comin, Filograsso, Day and Yáñez-Arancibia2013 and references cited therein). The first subregion, also called the ‘Campeche Bank’ is an extension of the Peninsula that is a vast and shallow continental shelf, which, like the terrestrial portion of the Yucatán Peninsula is composed of porous, Karst limestone of high permeability.This enables direct communication between continental and marine waters (Valle-Levinson et al., Reference Valle-Levinson, Mariño-Tapia, Enriquez and Waterhouse2011). The shallow environment of this subregion promotes important heat gain and evaporation, which, in the coastal lagoons and along the coastal environment in general, contributes to the development of warm and very saline waters that enter the GoM (Enriquez et al., Reference Enriquez, Mariño-Tapia, Jeronimo and Capurro-Filograsso2013). Also, being part of the GoM, tides of this subregion are predominantly diurnal (Tenorio-Fernandez et al., Reference Tenorio-Fernandez, Gomez-Valdes, Marino-Tapia, Enriquez, Valle-Levinson and Parra2016), with maximum tidal ranges that reach 0.6 m during tropical tides, and minimum ranges of only 0.1 m during equatorial tides, when the tide can be semi-diurnal, as long as it does not coincide with neap tides (Valle-Levinson et al., Reference Valle-Levinson, Enriquez and Mariño2022). The second subregion is different, as the water depth increases abruptly very close to the coast, where the intense oceanic Yucatán Current flows northward through the Yucatán Channel into the GoM. Tides in this subregion are semi-diurnal and also micro-tidal (<0.6 m range). Both regions are greatly influenced by the dominant trade winds (easterlies) and by substantial continental submarine fresh groundwater discharges. The north-eastern corner of the Yucatán Peninsula is a peculiar transition zone, which is influenced by both the Caribbean Sea and GoM. The region receives nutrient-rich upwelling water that arises from the Yucatán Current and moves onto the shallow Yucatán Shelf, interacting with the continental border (western boundary current) (Enriquez et al., Reference Enriquez, Mariño-Tapia and Herrera-Silveira2010; Reyes-Mendoza et al., Reference Reyes-Mendoza, Mariño-Tapia, Herrera-Silveira, Ruiz-Martínez, Enriquez and Largier2016). Linked to this transitional character of the Yucatán Peninsula, particular biological communities, including molluscs, are observed in near-coastal areas, including the coastal lagoons. Those communities differ from communities in the southern GoM to the west and in the Caribbean Sea to the east (Gonzalez et al., Reference Gonzalez, Chávez, de la Cruz and Torruco1991; Herrera-Silveira et al., Reference Herrera-Silveira, Comin, Filograsso, Day and Yáñez-Arancibia2013; Aguilar-Medrano & Vega-Cendejas, Reference Aguilar-Medrano and Vega-Cendejas2021).
Given the distinctive geographic characteristics of the northern Yucatán Peninsula and the paucity of mollusc research in the area, we undertook this study to evaluate mollusc community composition in the second largest coastal lagoon on the northern Yucatán Peninsula, i.e. the Río Lagartos coastal lagoon. Considering the marked spatial salinity gradient in this system, we also explored how mollusc species and functional groups are distributed with respect to salinity.
Materials and methods
Study area
The Río Lagartos coastal lagoon is located on the north-east coast of the Yucatán Peninsula (21°26′–21°38′N 87°30′–88°5W), Mexico, and is part of the Ría Lagartos Biosphere Reserve. This east–west-oriented lagoon is ~80 km long and has a total surface area of ~98 km2. The lagoon is divided into three hydrologically connected basins: Río Lagartos basin (mouth), Las Coloradas basin (middle) and El Cuyo basin (head (interior)) (Quesadas-Rojas et al., Reference Quesadas-Rojas, Enriquez and Valle-Levinson2021) (Figure 1A). It is generally shallow (~0.4–1.0 m) (Valdes & Real, Reference Valdes and Real2004; Quesadas-Rojas et al., Reference Quesadas-Rojas, Enriquez and Valle-Levinson2021), with some deeper locations, up to 3.5 m in channels or between zones (Quesadas-Rojas et al., Reference Quesadas-Rojas, Enriquez and Valle-Levinson2021). The lagoon is hypersaline throughout much of its length, with a gradient from the head near El Cuyo (>70) to the seaward region near Río Lagartos (33–38) (Herrera-Silveira & Ramírez-Ramírez, Reference Herrera-Silveira and Ramírez-Ramírez1998) (Figure 1B). Hypersaline conditions are a consequence of low rainfall (<500 mm/year), high evaporation (~2000 mm/year), absence of overland freshwater inputs, long residence times, and physical/geomorphological characteristics (Valdes & Real, Reference Valdes and Real2004; Peralta-Meixueiro & Vega-Cendejas, Reference Peralta-Meixueiro and Vega-Cendejas2011).

Fig. 1. (A) Location of the Río Lagartos coastal lagoon on the northern Yucatán Peninsula, Mexico. The extents of the three main basins of the system are shown (Río Lagartos basin (mouth), Las Coloradas basin (middle) and El Cuyo basin (head)). Black dots indicate sites where molluscs were collected, open squares indicate sites without molluscs and letters denote the season when molluscs were collected (N: cool Nortes season (February sampling), R: rainy season (September/October sampling), D: warm and dry season (late May sampling)). (B) Heat map of the salinity values per station (shown at the top) and season (D: warm and dry, N: cool Nortes, R: rainy) (shown on the left).
Field sampling and data collection
Sampling strategy
Samples were collected in the Río Lagartos coastal lagoon system (Figure 1A) as part of the Salinity Gradient Energy project of the Centro Mexicano de Innovación en Energía Océano (CEMIE Océano). Samples were obtained from 16 stations distributed along the lagoon, in the three main basins of the system: Río Lagartos basin to the mouth, in the west (stations: 3, 6, 9, 12, 15), Las Coloradas basin, in the middle (stations: 20, 21, 22, 23, 25) and El Cuyo basin to the head of the lagoon, in the east (stations: 27, 29, 30, 32, 35, 38) (Figure 1A; Table 1). Spatial distribution of the stations was based on previous studies of abiotic and biotic variables, which indicated that the three basins can be considered environmentally and biologically distinct (Ortegón-Aznar et al., Reference Ortegón-Aznar, González-González and Granados2001; Valdes & Real, Reference Valdes and Real2004; Vega-Cendejas & Hernández De Santillana, Reference Vega-Cendejas and Hernández De Santillana2004; Peralta-Meixueiro & Vega-Cendejas, Reference Peralta-Meixueiro and Vega-Cendejas2011; Dávila-Jiménez et al., Reference Dávila-Jiménez, Papiol, Hernández-Alcántara, Enriquez, Sauma-Castillo and Chiappa-Carrara2019). The lagoon was sampled during three periods that coincided with the end of the three seasons in the region: the rainy season (September/October 2017), the cool Nortes season (February 2018) and the warm, dry season (May 2018) (Table 1). This was done to capture seasonal differences in the mollusc fauna throughout the year. Samples were collected at water depths ranging from 0.5–1.5 m.
Table 1. Sampling locations where molluscs were collected in the hypersaline Río Lagartos coastal lagoon, Yucatán Peninsula, Mexico

Survey letters ‘RL’ indicate Río Lagartos and survey numbers indicate the year of sampling and month when the sampling survey started. Site numbers are as indicated in Figure 1A and locations are designated by latitude (north) and longitude (west). Date is collection date (year-month-day). Season of collection is indicated by R: rainy season (September/October sampling), N: cool Nortes season (February sampling), and D: warm and dry season (late May sampling). Basin of the lagoon system (RL: Río Lagartos (mouth), CO: Las Coloradas (middle), CU: El Cuyo (head)), and value of salinity in Practical Salinity Units (PSU) also indicated.
Mollusc sampling
Molluscs were collected using three methods. Samples of macrobenthic molluscs were obtained using a standard Ponar dredge (9″ × 9″ or 22.9 × 22.9 cm; 0.052 m2 sampling area), or a cylindrical PVC core tube (6″ diameter; 0.018 m2 sampling area), the latter in the event that the substrate was too hard and the dredge did not penetrate the sediment. Duplicate samples were obtained at each station, and sediments were sieved through a 500-μm mesh to separate the macrofauna. Megabenthic molluscs were collected using a 40-m-long and 1-m-high beach seine with half-inch mesh size, covering an area of 400 m2. Molluscs on the roots of red mangrove (Rhizophora mangle Linnaeus, 1753) were also obtained in some cases, by gently scraping them from the root surface over a 20 × 20 cm2 area, at mid-depth in the water column. Details about the sampling locations and gear used are found in Supplementary Material 1. Specimens from one replicate at each station were initially anaesthetized with magnesium chloride, and then preserved in 4% formaldehyde. The other replicate was frozen for future chemical analyses. In the laboratory, the formaldehyde samples were transferred to 70% ethanol, after which all molluscs were sorted and identified, usually to species level, under a stereo- or a compound microscope.
Taxonomic classification largely followed Bouchet et al. (Reference Bouchet, Rocroi, Bieler, Carter and Coan2010) for Bivalvia, and Bouchet et al. (Reference Bouchet, Rocroi, Hausdorf, Kaim, Kano, Nützel, Parkhaev, Schrödl and Strong2017) for Gastropoda, though some classifications were updated using Mikkelsen & Bieler (Reference Mikkelsen and Bieler2008), Redfern (Reference Redfern2013) and Tunnell et al. (Reference Tunnell, Andrews, Barrera and Moretzsohn2010). Molluscs were identified to the lowest possible taxonomic level, using multiple sources (García-Cubas, Reference García-Cubas1981; García-Cubas & Reguero, Reference García-Cubas and Reguero2007; Mikkelsen & Bieler, Reference Mikkelsen and Bieler2008; Tunnell et al., Reference Tunnell, Andrews, Barrera and Moretzsohn2010; Espinosa et al., Reference Espinosa, Ortea, Sánchez and Gutiérrez2012; Redfern, Reference Redfern2013), and photographed. The term specimen generally refers to live-collected animals with soft parts intact. Bivalve shells are described as ‘paired’, and only articulated individuals were photographed. Only for the species of gastropod Cerithium atratum photographs were obtained from a dead specimen with a sipunculid worm inside, but for numerical analyses only live animals were considered. Each specimen was assigned a catalogue number and deposited in the ‘Colección de Moluscos de la Península de Yucatán’ (CMPY), Unidad Multidisciplinaria de Docencia e Investigación Campus Sisal, Universidad Nacional Autónoma de México.
Salinity measurement
At each station, salinity was measured in the water column at depths between 0.15–0.35 m, using a multiparameter Aquaprobe AP-5000. A refractometer was used to measure salinity when values exceeded 60, and a Baume hydrometer was used when salinity was >80.
Sediment sampling
Sediments were sampled with the same dredge or PVC core barrel used for biological sampling and collected material was stored at −20°C for grain-size analysis. In the laboratory, sediments were dried at 60°C to a constant weight, and later, proportions of gravel (>2 mm), sand (0.063–2 mm) and silt/clay (<0.063 mm) (Wentworth, Reference Wentworth1922) were obtained by sieving sediments with a sieve agitator for 20 min.
Submersed vegetation
The macroscopic vegetation collected in each macrobenthos sample was separated from the sediment after thorough inspection under the microscope to separate the organisms. Wet weights of the vegetation and sediments were obtained after drying with blotting paper, and the gravimetric contribution of vegetation (macroalgae and seagrasses) to the whole sample (i.e. vegetation and sediments) was calculated as a proxy for the amount of vegetation in the sample.
Data analysis
Community composition analysis
A sample presence-absence matrix was constructed. Presence of the species in each basin and during each sampling period was examined and the total number of species was obtained. Organisms were classified according to their taxonomic class (Bivalvia or Gastropoda), habitat (infaunal or epifaunal) and feeding guild (suspension feeders, carnivores, deposit consumers or grazers), following the classification scheme of García-Cubas (Reference García-Cubas1981) and García-Cubas & Reguero (Reference García-Cubas and Reguero2007), and the number of species in each group was determined. These three classifications were related in an alluvial diagram with the online software Raw graphs 2.0.
Species vs salinity analysis
Samples were classified into two groups based on salinity (euhaline (30–40) and hyperhaline (>40)), following the classification of Wingard et al. (Reference Wingard, Hudley, Bay and National2012), and the salinity range occupied by each species was explored. Next, species were classified into two groups based on salinity tolerance, i.e. stenohaline (occurring in <20% of the total salinity range in the lagoon) and euryhaline (occurring in >20% of the total salinity range in the lagoon). The per cent contribution to the total number of species and the frequency of occurrence (FO% = number of samples in which a class was present/total number of samples × 100) of each taxonomic class, habitat group and feeding guild were calculated for the euhaline and hyperhaline environments. Mean values and standard deviations of the different sediment grain-size classes and of the submersed vegetation gravimetric percentages were also estimated for both environments.
Species accumulation curves were constructed for each salinity environment with interpolation-extrapolation of Hill Numbers of order q: species number (q = 0) to compute diversity estimates and the associated 95% (0.95) confidence intervals for the two groups, based on salinity. Analyses were performed using the online package iNEXT (Chao et al., Reference Chao, Ma and Hsieh2016). A data summary is provided in Supplementary Material 2.
Results
Taxonomic composition
A total of 2598 mollusc specimens from the Río Lagartos coastal lagoon, representing 39 species (Table 2), 34 genera, 23 families (Figures 2–5), and two classes, were enumerated. Molluscs were found at seven stations in the dry season, six stations in the cold (Nortes) season and 11 stations in the rainy season. Species Carditamera floridana, Meioceras nitidum, Modulus modulus and Crepidula convexa were present during all three seasons (Table 2). Nine species were collected during the cold season, 22 species in the rainy season and 28 species in the warm dry season. Thirty-seven species were collected in the Río Lagartos basin (mouth) (RL), six species in the Las Coloradas basin (middle) (CO) and five species in the El Cuyo basin (head) (CU) (Table 2). The most ubiquitous species were Prunum apicinum (Menke, 1828), encountered at 14 stations, Anomalocardia auberiana, encountered at nine stations, and Meioceras nitidum (Stimpson, 1851), Angulus paramerus (Boss, 1964), Modulus modulus (Linnaeus, 1758), Crepidula convexa (Say, 1822) and Carditamera floridana (Conrad, 1838), encountered at eight stations. Thirty-three species were collected with the dredge, 12 species with the beach seine and four species with the cylindrical PVC tube. Twenty species (51%) belonged to the class Gastropoda, and 19 (49%) to the Bivalvia (Figure 6). Twenty-two species (56%) were epifaunal and 17 (44%) were infaunal (Table 2, Figure 6). Nine species (23%) were carnivores, 14 (36%) were suspension feeders, seven (18%) were deposit consumers and nine (23%) grazers (Table 2, Figure 6).

Fig. 2. Mytilidae: Brachidontes exustus: (A, B). Musculus lateralis: (C–D). Lucinidae: Anodontia alba: (E–G). Ctena orbiculata: (H–J). Lucinisca nassula: (K–M). Parvilucina crenella: (N–P). Carditidae: Carditamera floridana: (Q–S). Crassatellidae: Crassinella sp.: (T). Lasaeidae: Lasaeidae sp.: (U, V). Tellinidae: Angulus merus: (W–Y) Scale bars = 1 mm.
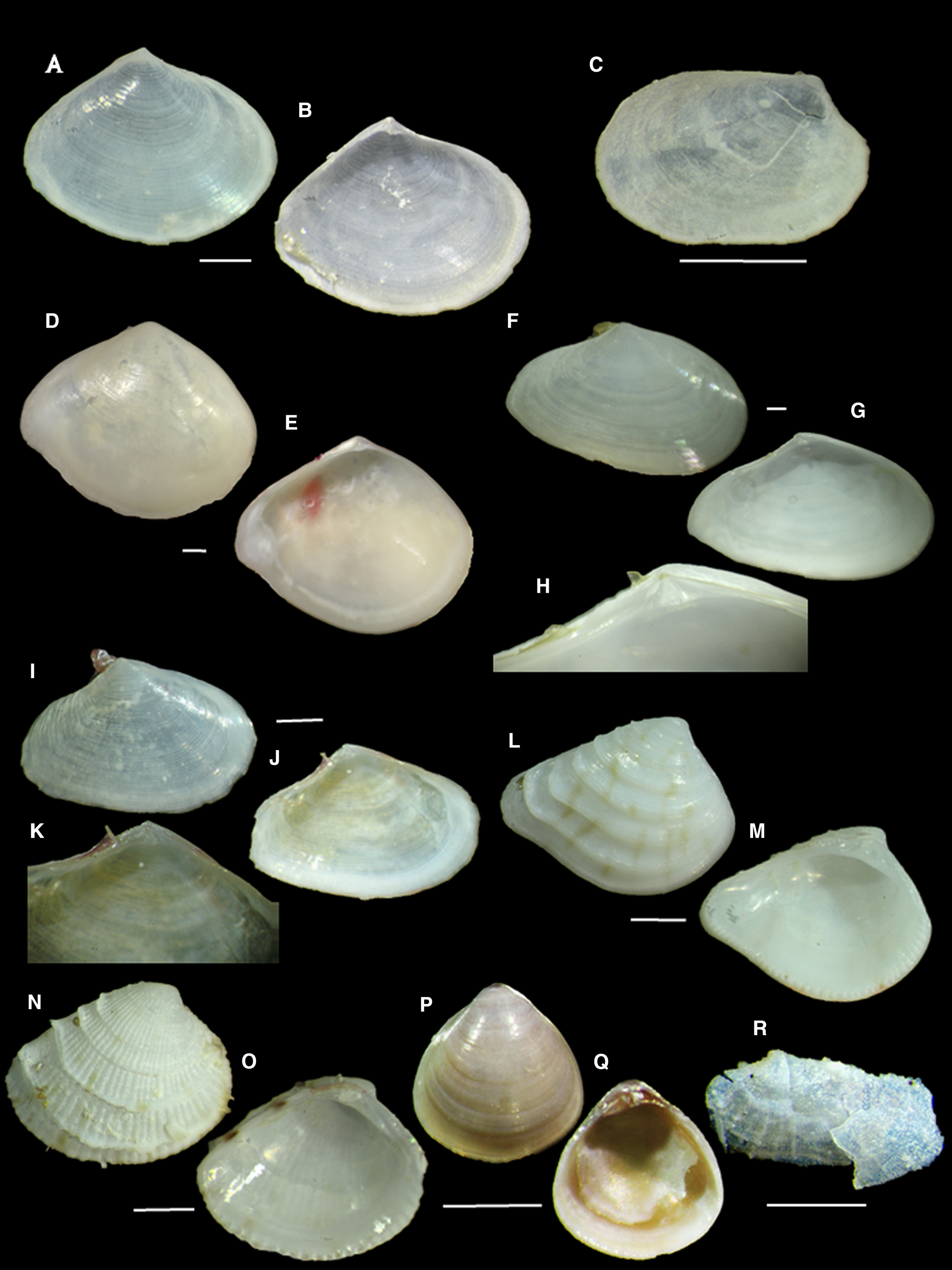
Fig. 3. Tellinidae: Angulus paramerus: (A, B). Angulus sybariticus: (C). Macoma cerina: (D, E). Macoma tenta: (F–H). Merisca sp.: (I–K). Veneridae: Anomalocardia auberiana: (L, M). Chione cancellate: (N, O). Parastarte triquetra: (P, Q). Lyonsiidae: Lyonsia sp.: (R). Scale bars = 1 mm.

Fig. 4. Phasianellidae: Eulithidium adamsi: (A, B). Cerithiidae: Cerithiidae sp.: (C, D). Cerithium atratum: (E, F) (with Sipuncula). Cerithium eburneum: (G). Cerithium lutosum: (H, I). Modulidae: Modulus modulus: (J, K). Vermetidae: Vermetidae sp.: (L, M). Caecidae: Caecum donmoorei: (N, O). Meicoceras nitidum: (P, Q). Zebinidae: Zebina browniana: (R–T). Calyptraeidae: Crepidula convexa: (U–W). Marginellidae: Granulina sp.: (X–Z). Scale bars = 1 mm.

Fig. 5. Marginellidae: Prunum apicinum: (A–E). Columbellidae: Astyris antares: (F, G). Melongenidae: Melongena corona (H, I) (scale bar = 5 mm). Nassariidae: Nassarius vibex: (J). Murchisonellidae: Henrya henryi: (K–M). Bullidae: Bulla occidentalis: (N–P). Haminoeidae: Haminoea succinea: (Q, R). Pyramidellidae: Odostomia laevigata: (S–U). Scale bars = 1 mm.

Fig. 6. Alluvial plot depicting the relationship between classes and species for two different functional traits: habitat and feeding guild. The species numbers are provided for each category.
Table 2. Species presence by season (N: cool and dry Nortes season (February sampling), R: rainy season (September/October sampling), D: warm and dry season (late May sampling)), basin (RL – Río Lagartos (mouth), CO – Las Coloradas (middle), CU – El Cuyo (head)), and sampler used (dredge, beach seine and PVC tube). Feeding guild (SF: suspension feeder, DC: deposit consumers, G: grazer, C: carnivore) and habitat (E: epifaunal, I: infaunal) of each species also indicated

Molluscs and salinity in the Río Lagartos coastal lagoon
The Río Lagartos coastal lagoon is inhabited by both stenohaline (27 species; 69%) and euryhaline (12 species; 31%) species (Figure 7). Stenohaline species displayed lower occurrence (<20%), and some species were collected exclusively under euhaline (15 species) or hypersaline conditions (6 species). Twelve species tolerate a broad salinity range (euryhaline). The species distributed in the largest salinity range were: Melongena corona (37–78), Anomalocardia auberiana (34–58), Meioceras nitidum (36–74) and Odostomia laevigata, which was collected at only two stations with very different salinity (35 and 78).

Fig. 7. Occurrence of mollusc species vs salinity. Ranges for euhaline (marine, 30–40 PSU) and hyperhaline environments (40–80 PSU) are indicated at the bottom of the figure. Salinity tolerance groups, i.e. stenohaline and euryhaline, are shown on the right. Terminology follows Wingard et al. (Reference Wingard, Hudley, Bay and National2012).
Abiotic characteristics and fauna composition differed between the two salinity environments (Table 3). In the euhaline sites, sediments were mostly sandy, and gravel and silt/clay were notably scarce (<10%) (Table 3). Percent submersed vegetation was 15.25 ± 29%. Thirty-five species were collected in euhaline sites. Gastropoda and epifaunal molluscs occurred in all stations (FO = 100%). With respect to feeding guild, suspension feeders and grazers (FO = 79%) and carnivores (FO = 68%) appeared in more than half the samples. Suspension feeders were the most diverse group (40% of the species), followed by carnivores and grazers, each representing 23% of the species. In the hyperhaline sites, sediments were again mostly sandy, but the percentage contributions of gravel (7.8%) and silt/clay (11.3%) were greater than in the euhaline sites, and the sediments were more variable (Table 3). The percentage of submersed vegetation was lower (4.9 ± 7.9%). We found 22 species associated with hyperhaline sites, and of those, infauna (FO = 79%) and the class Bivalvia (FO = 79%) were distributed extensively in the samples. With respect to feeding guilds, suspension feeders appeared in more than half of the samples (FO = 64%), and the rest of the guilds were distributed in <50% of the samples. The most diverse groups in the hypersaline environments were suspension feeders (36% of the species) and carnivores (32% of the species).
Table 3. Salinity ranges, number of sites (including samples in each season), proportions of sediment size-classes (gravel, sand, silt/clay) and submersed vegetation gravimetric contribution to the surface sediment samples (mean value ± SD). The total number of species (S) per environment and species number range per site (in parenthesis) in the environment also shown. The percentage contribution to the total number of species (S) and frequency of occurrence (FO% = number of samples in which a class was present/total number of samples × 100) in the mollusc groups by class (Gastropoda and Bivalvia), habitat (epifauna and infauna), or feeding guild (suspension feeders, carnivores, deposit consumers and grazers) in the euhaline and hyperhaline environments of the Río Lagartos coastal lagoon are on the bottom

For comparison of diversity between the euhaline and hyperhaline environments, sample-size-based sampling curves were constructed, using reference sample sizes of 33 and 24 (considering each replicate), respectively. Extrapolation was carried out to >40 samples in both cases (Figure 8A). In all cases, sample completeness was <1 (0.8 for the hyperhaline environment and 0.7 for the euhaline environment) (Figure 8B), which indicates a deficit in the detection of species of about 20%. Comparisons of coverage-based sampling curves, up to a base coverage, revealed higher diversity than expected in euhaline environments (Figure 8C).

Fig. 8. Interpolation and extrapolation curves of mollusc species in samples from the Río Lagartos coastal lagoon under euhaline (red line) and hyperhaline (blue lines) conditions. (A) Species accumulation curves based on the occurrence of species . (B) Sample-coverage accumulation curve . (C) Sample completeness curves, linking curve 8A and 8B. The solid lines represent interpolation, whereas the dashed lines depict extrapolation.
Discussion
Taxonomic composition
This work updates and expands upon previous mollusc studies in the Río Lagartos coastal lagoon. We encountered 37 species in the Río Lagartos basin (mouth), six in the Las Coloradas basin (middle) and five in the El Cuyo basin (head). The numbers differ substantially from those obtained by Gonzalez et al. (Reference Gonzalez, Chávez, de la Cruz and Torruco1991), who reported the presence of 71 species in the Río Lagartos basin, four in the Las Coloradas basin, and one in the El Cuyo basin. Furthermore, only nine of the species found in this study overlap with those reported by Gonzalez et al. (Reference Gonzalez, Chávez, de la Cruz and Torruco1991) (Anodontia alba, Carditamera floridana, Chione cancellata, Anomalocardia auberiana, Modulus modulus, Cerithium atratum, C. eburneum, Melongena corona and Bulla occidentalis), all of which were collected from the Río Lagartos basin, only one from the Las Coloradas basin (Melongena corona) and none from the El Cuyo basin. The difference in number and composition of species between the two studies may be a consequence of several factors, some related to changes in the environmental conditions, and others related to sampling and sample-processing methods. Regarding the environment, it is unlikely that the effects of recent climate change, which have been detected on the Yucatán Peninsula during the last 60 years (Carnero-Bravo et al., Reference Carnero-Bravo, Sanchez-Cabeza, Ruiz-Fernández, Merino-Ibarra, Corcho-Alvarado, Sahli, Hélie, Preda, Zavala-Hidalgo, Díaz-Asencio and Hillaire-Marcel2018; Andrade-Velázquez et al., Reference Andrade-Velázquez, Medrno-Pérez, Montero-Martínez and Alcudia-Aguilar2021), affected the mollusc community composition inside the lagoon, as detected long-term variations are small compared with short-term (diel, fortnightly or seasonal) variations that occur in the waterbody. For instance, no directional change in lagoon salinity has been detected during the last 40 years (Wojtarowski et al., Reference Wojtarowski, Matrínez, Silva, Vázquez, Enriquez, López-Portillo, García-Franco, MacGregor-Fors, Lara-Domínguez and Lithgow2021). Given the rapid population growth and increased human activities around the lagoon during that same period, it is more plausible that the differences in faunal composition between the two studies are related to greater recent anthropogenic activities. For example, microplastics have been found in large quantities in recent lagoon sediments, and have been linked to human activity in the area (Quesadas-Rojas et al., Reference Quesadas-Rojas, Enriquez and Valle-Levinson2021). Nevertheless, interannual differences in abiotic conditions related to, for example, changes in precipitation or impacts of individual extreme events such as tropical storms and hurricanes (De la Barreda et al., Reference De la Barreda, Metcalfe and Boyd2020), or longer-term climate variations (e.g. El Niño–Southern Oscillation; Fichez et al., Reference Fichez, Archundia, Grenz, Douillet, Gutiérrez Mendieta, Origel Moreno, Denis, Contreras Ruiz Esparza and Zavala-Hidalgo2017), may also be responsible for such fauna differences between the two studies. Although all these explanations are plausible, we cannot rule out the possibility that differences between the two studies are attributable to sampling and sample-processing differences. Those methodological differences relate to: (1) the timing of sampling during the year, as suggested by the seasonal changes in species richness detected in our results, with the highest number of species (28) found in the dry season; (2) the types of samplers used, as the selectivity of each sampler is related to the net mesh size and the mode of operation (Raz-Guzman & Grizzle, Reference Raz-Guzman, Grizzle, Short and Coles2001) – in this study, the dredge retained more species (33) than did the other samplers (Supplementary Material 3); and (3) the use of both empty shells and live specimens in counts in the previous study. Determination of the true cause(s) of long-term temporal patterns in mollusc fauna composition in the lagoon would require regular monitoring and use of standardized methods, which are expensive and difficult to sustain. Such large-scale efforts, which use standardized protocols (MBON Pole to Pole, 2019; Obst et al., Reference Obst, Exter, Allcock, Arvanitidis, Axberg, Bustamante, Cancio, Carreira-Flores, Chatzinikolaou, Chatzigeorgiou, Chrismas, Clark, Comtet, Dailianis, Davies, Deneudt, de Cerio, Fortič, Gerovasileiou, Hablützel, Keklikoglou, Kotoulas, Lasota, Leite, Loisel, Lévêque, Levy, Malachowicz, Mavrič, Meyer, Mortelmans, Norkko, Pade, Power, Ramšak, Reiss, Solbakken, Staehr, Sundberg, Thyrring, Troncoso, Viard, Wenne, Yperifanou, Zbawicka and Pavloudi2020; Ashton et al., Reference Ashton, Freestone, Duffy, Torchin, Sewall, Tracy, Albano, Altieri, Altvater, Bastida-Zavala, Bortolus, Brante, Bravo, Brown, Buschmann, Buskey, Barrera, Cheng, Collin, Coutinho, De Gracia, Dias, DiBacco, Flores, Haddad, Hoffman, Erquiaga, Janiak, Campeán, Keith, Leclerc, Lecompte-Pérez, Longo, Matthews-Cascon, McKenzie, Miller, Munizaga, Naval-Xavier, Navarrete, Otálora, Palomino-Alvarez, Palomo, Patrick, Pegau, Pereda, Rocha, Rumbold, Sánchez, Sanjuan-Muñoz, Schlöder, Schwindt, Seemann, Shanks, Simoes, Skinner, Suárez-Mozo, Thiel, Valdivia, Velez-Zuazo, Vieira, Vildoso, Wehrtmann, Whalen, Wilbur and Ruiz2022), can be employed to detect shifts in community composition going forward. Such programmes, however, cannot shed light on past community changes. To document such past changes, it will be necessary to undertake palaeoecological studies, like those that have been done to examine changes in marine fauna communities during the last century, in both coastal (Armenteros et al., Reference Armenteros, Díaz-Asencio, Fernández-Garcés, Hernández, Helguera-Pedraza, Bolaños-Alvarez, Agraz-Hernández and Sanchez-Cabeza2016) and open-ocean contexts (Cartes et al., Reference Cartes, Schirone, Barsanti, Belbono, Martínez-Aliaga and Lombarte2017, Reference Cartes, Díaz-Viñolas, Schirone, Lombarte, Barsanti, Delbono, Salas, Gofas, Serrano and Santos-Echeandía2022).
In any case, the large disparity in the diversity between the two studies, together with the revealed deficit in the detection of species in this study, as seen in the species accumulation curves, reinforces the idea that, despite the great sampling effort, the Río Lagartos coastal lagoon has greater mollusc diversity, and likely spatio-temporal heterogeneity, than reported in this or previous studies. This highlights the lack of information from the region that will be necessary to achieve solid ecological baseline knowledge (Guerra-Castro et al., Reference Guerra-Castro, Hidalgo, Castillo-Cupul, Muciño-Reyes, Noreña-Barroso, Quiroz-Deaquino, Mascaro and Simoes2020).
All the species found in the Río Lagartos coastal lagoon have been reported previously from other lagoons around the Gulf of Mexico (Figure 9) and 22 species were reported earlier from the Caribbean (García-Cubas & Reguero, Reference García-Cubas and Reguero2004, Reference García-Cubas and Reguero2007; Rosenberg et al., Reference Rosenberg, Moretzsohn and Garcia2009; Turgeon et al., Reference Turgeon, Lyons, Mikkelsen, Rosenberg and Moretzsohn2009). The high number of shared species between the Gulf of Mexico and the Caribbean is attributable to the dispersal of larvae by the Yucatán Current. Larvae may be carried from the Caribbean onto the continental shelf, particularly by upwelling events that occur seasonally, typically from April to September (Sanvicente-Añorve et al., Reference Sanvicente-Añorve, Zavala-Hidalgo, Allende-Arandía and Hermoso-Salazar2014; Reyes-Mendoza et al., Reference Reyes-Mendoza, Mariño-Tapia, Herrera-Silveira, Ruiz-Martínez, Enriquez and Largier2016; Kurczyn et al., Reference Kurczyn, Duran, Beier and Souza2021). Once in the northern coastal region of the Yucatán Shelf, the main current is directed westward along the coast mainly by winds, and enhanced by westward pulses of the Yucatán Current, which flows to the north (Enriquez et al., Reference Enriquez, Mariño-Tapia, Jeronimo and Capurro-Filograsso2013). The Trade Winds (easterlies) are locally deflected southward by intense diurnal onshore breezes, which keep the westward-flowing waters close to the coast. This transport favours connectivity among populations (Herrera-Silveira et al., Reference Herrera-Silveira, Comin, Filograsso, Day and Yáñez-Arancibia2013).

Fig. 9. Comparison of molluscs recorded in the Río Lagartos coastal lagoon (present study) and in other coastal lagoons around the Gulf of Mexico, Mexico (García-Cubas, Reference García-Cubas1963, Reference García-Cubas1981; García-Cubas et al., Reference García-Cubas, Escobar de la Llata, Gonzalez Ania and Reguero1990, Reference García-Cubas, Reguero and Elizarrarás1992; García-Cubas and Reguero, Reference García-Cubas and Reguero1990, Reference García-Cubas and Reguero1995; Reguero and García-Cubas, Reference Reguero and García-Cubas1993, Reference Reguero and García-Cubas1994, Reference Reguero and García-Cubas1989, Reference Reguero and García-Cubas1991b, Reference Reguero and García-Cubas1991a; De Jesús-Carrillo et al., Reference De Jesús-Carrillo, Ocaña, Hernández-Ávila, Mendoza-Carranza, Sánchez and Barba-Macías2020).
Abiotic and biotic patterns
This study demonstrates that mollusc species richness in the Río Lagartos coastal lagoon is influenced by salinity. Species richness was higher under euhaline than hypersaline conditions, and no animals were collected at salinities >78. A decrease in species richness above hypersaline values in aquatic environments is common (Deaton, Reference Deaton2019) and in the Río Lagartos coastal lagoon it is reflected by the substantially lower numbers of species in the Las Coloradas (middle) and El Cuyo (head) basins. Such a pattern was also detected by Gonzalez et al. (Reference Gonzalez, Chávez, de la Cruz and Torruco1991), and, in fact, the authors reported that the Las Coloradas and El Cuyo basins behaved as entities independent from the Río Lagartos basin, in terms of species richness.
Experimental studies on salinity tolerances of mollusc species and the mechanisms that underlie salinity limitation are notably scarce and mainly limited to species of economic interest (Calabrese & Davis, Reference Calabrese and Davis1970; Nell & Gibbs, Reference Nell and Gibbs1986; Deaton et al., Reference Deaton, Derby, Subhedar and Greenberg1989; Yang et al., Reference Yang, Zhong, Zhao, Wu, Du, Liu, Zhang, Yan and Huang2018), and a few others (Berger & Kharazova, Reference Berger and Kharazova1997; Przeslawski, Reference Przeslawski2004). Most of those studies considered salinities less than hypersaline, and little or nothing is yet known about the osmoregulatory physiology of hypersaline molluscs (Berger & Kharazova, Reference Berger and Kharazova1997; Deaton, Reference Deaton2019). Khlebovich & Kondratenkov (Reference Khlebovich and Kondratenkov1973) reported the high-salinity acclimation threshold for the snail Hydrobia ulvae at values between 75 and 80, and for other macrobenthic invertebrates such as polychaetes, survival was restricted to salinity values <70. Thus, absence of molluscs at salinities >78 may indicate that is a threshold value for this taxonomic group. Below 78, bivalves were more diverse and widespread under hypersaline conditions than were gastropods, the latter of which prevailed under euhaline conditions. Bivalves can withstand stressful conditions and respond to changes in environmental salinity by closing their shells (Vlašić et al., Reference Vlašić, Glavić, Bolotin, Hrustić and Radić2018). In the case of gastropods, extreme values of salinity and temperature slow development and/or increase embryonic mortality (Przeslawski, Reference Przeslawski2004). On the other hand, the low incidence of gastropods could be a consequence of a low availability of food and reduced feeding activity under stressful conditions.
The bivalve Anomalocardia auberiana and the gastropods Meioceras nitidum and Melongena corona were among the species distributed across the largest salinity ranges and the first two were among the most ubiquitous species in the Río Lagartos coastal lagoon. The large salinity tolerance ranges and the capacity to live under very high salinities, up to 58 for A. auberiana, 74 for M. nitidum and 78 for M. corona, explains their widespread distribution in the lagoon. Tolerance of hypersaline conditions was reported for A. auberiana in the Baffin Bay estuary (south Texas, USA) (Rubio et al., Reference Rubio, Ajemian, Stunz, Palmer, Lebreton and Beseres Pollack2018), and Melongena spp. inhabit different ecosystems (i.e. shallow, low-energy embayments, lagoons, salt marshes, mangrove swamps and oyster bars) (Hayes, Reference Hayes2003), which suggests a wide salinity tolerance of the genus. Instead, M. nitidum has usually been found in shallow habitats where the salinity is close to that of the ocean (Lester-Coll, Reference Lester-Coll2017). This suggests strong physiological plasticity and may point to high capacity for acclimation, as reported for other marine snails (Berger & Kharazova, Reference Berger and Kharazova1997). A similar yet less marked tolerance for high salinity (to 50 PSU) caused by exposure to high salinity values, can be deduced for the most frequently encountered mollusc species in the Río Lagartos coastal lagoon, the gastropod Prunum apicinum. This species was previously found inhabiting environments with salinity up to 40, with a preferred range of about 15–25 (Cain, Reference Cain1972; Roessler & Tabb, Reference Roessler and Tabb1974; Tampa Bay Water & Janicki Environmental Inc., 2003; Jiménez-Ramos & Acosta-Balbás, Reference Jiménez-Ramos and Acosta-Balbás2020). The very high and unstable salinity conditions that prevail in the Río Lagartos coastal lagoon probably favour the adaptation of species, which represents an adaptive advantage, given the predicted salinity increase associated with ongoing climate change (Curry et al., Reference Curry, Dickson and Yashayaev2003; Boyer et al., Reference Boyer, Levitus, Antonov, Locarnini and Garcia2005). This is especially relevant in this type of lagoon, where limited freshwater inputs and high evaporation rates drive salinity increases.
Salinity may not be the only factor responsible for the spatial distribution patterns of molluscs. Rather, substrate type, sediment grain size, hydrodynamics and salinity likely interact to create such patterns. Furthermore, the effects of salinity on molluscs may be direct, by acting on their osmotic and ionic equilibrium, or indirect, by affecting the distribution of other factors that in turn regulate mollusc distribution. For example, in the Río Lagartos coastal lagoon, the distribution of submersed vegetation, which serves as habitat or a food source for many macrobenthic taxa, is strongly influenced by salinity (Ortegón-Aznar et al., Reference Ortegón-Aznar, González-González and Granados2001). In this sense, higher species richness and frequency of occurrence of gastropods in the euhaline environment may be associated with the greater amount of submersed vegetation. The macrophytes provide a range of habitat types for epifaunal organisms and food for grazers, both functional groups being represented by gastropods in the Río Lagartos coastal lagoon. Accordingly, the widespread distribution of Meioceras nitidum, an epifaunal grazer, is enhanced by both its euryhaline character and a considerable capacity to inhabit a diverse array of substrates, as live specimens have been found on mangrove roots, algae from the intertidal to subtidal zone, and seagrass beds (Tunnell et al., Reference Tunnell, Andrews, Barrera and Moretzsohn2010; Redfern, Reference Redfern2013; Pereira Costa et al., Reference Pereira Costa, Setubal Pires-Vanin and Barbosa Lima2021). Conversely, higher occurrence and diversity of bivalves, which are predominantly infaunal, in the hypersaline environments, may be favoured by lower amounts of vegetation on the bottom, and the presence of a broader array of sediment grain sizes that are suitable for a large number of burrowing species (Alexander et al., Reference Alexander, Stanton and Dodd1993), or for suspension and deposit feeders.
Complex hydrodynamics and geomorphology of the lagoon favour high sediment heterogeneity. This may explain the presence of large denuded areas, even in the euhaline sites, which may trigger the spread of A. auberiana, an opportunistic species with a high capacity to rapidly invade newly available habitat, and soon after appear in large numbers (Grizzle, Reference Grizzle1984; Kuk-Dzul et al., Reference Kuk-Dzul, Gold-Bouchot and Ardisson2012). Although tides have little influence throughout the entire lagoon, except near the mouth of the Río Lagartos basin, the local dynamics are important in the middle and upper basins because of their sizes, depths and processes therein. Possessing vast shallow areas, these basins are subject to the effects of wind and waves, which mix the whole water column and enhance resuspension of sediment particles throughout the area, providing food for the suspension feeders that dominate the lagoon's mollusc community.
Most of the stenohaline species in this study were rare, having been captured at only one or two stations. Although the sampling limitations mentioned previously cannot be ruled out as having caused the low recovery of these species (Lyons et al., Reference Lyons, Brigham, Traut and Schwartz2005), low salinity tolerance, together with impaired dispersal or colonizing ability linked to the small-scale environmental heterogeneity of the system also seems a plausible explanation (Cao et al., Reference Cao, Larsen and Thorne2001). For example, Parastarte triquetra was found at only two stations and in a narrow salinity range, consistent with the very localized distribution of the species recorded in other systems (Grizzle, Reference Grizzle1984; Morelos-Villegas et al., Reference Morelos-Villegas, Condal and Ardisson2018). This species has been correlated with high values of trichlorobenzene in the Yucatán Peninsula coastal lagoons, and in this study it appeared at stations where high levels of pollution were reported previously (Kuk-Dzul et al., Reference Kuk-Dzul, Gold-Bouchot and Ardisson2012).
This work revealed that the current state of knowledge regarding the abiotic and biotic controls on mollusc distributions in the eastern GoM is poor. Information from this study serves as a starting point (‘baseline’) for studies of future impacts on the Río Lagartos coastal lagoon, for example, changes in salinity regime and consequent redistribution of organisms. Our study also raises an important question: what factor or factors account for the lower (45.1%) number of mollusc species found in this study, relative to the number encountered in a similar study carried out almost four decades ago? The relative roles of natural variation and anthropogenic activities on mollusc diversity changes remain to be distinguished and are crucial to understand the potential effects of ongoing global change on mollusc diversity. This question needs to be addressed cautiously, as factors independent from environmental variables, such as the types of samplers used, sampling intensity and time of the year, and high numbers of rare species, can all affect the number of species collected. We encourage colleagues who undertake similar future studies to collaborate with large-scale networks that use standardized protocols (MBON Pole to Pole, 2019; Obst et al., Reference Obst, Exter, Allcock, Arvanitidis, Axberg, Bustamante, Cancio, Carreira-Flores, Chatzinikolaou, Chatzigeorgiou, Chrismas, Clark, Comtet, Dailianis, Davies, Deneudt, de Cerio, Fortič, Gerovasileiou, Hablützel, Keklikoglou, Kotoulas, Lasota, Leite, Loisel, Lévêque, Levy, Malachowicz, Mavrič, Meyer, Mortelmans, Norkko, Pade, Power, Ramšak, Reiss, Solbakken, Staehr, Sundberg, Thyrring, Troncoso, Viard, Wenne, Yperifanou, Zbawicka and Pavloudi2020; Ashton et al., Reference Ashton, Freestone, Duffy, Torchin, Sewall, Tracy, Albano, Altieri, Altvater, Bastida-Zavala, Bortolus, Brante, Bravo, Brown, Buschmann, Buskey, Barrera, Cheng, Collin, Coutinho, De Gracia, Dias, DiBacco, Flores, Haddad, Hoffman, Erquiaga, Janiak, Campeán, Keith, Leclerc, Lecompte-Pérez, Longo, Matthews-Cascon, McKenzie, Miller, Munizaga, Naval-Xavier, Navarrete, Otálora, Palomino-Alvarez, Palomo, Patrick, Pegau, Pereda, Rocha, Rumbold, Sánchez, Sanjuan-Muñoz, Schlöder, Schwindt, Seemann, Shanks, Simoes, Skinner, Suárez-Mozo, Thiel, Valdivia, Velez-Zuazo, Vieira, Vildoso, Wehrtmann, Whalen, Wilbur and Ruiz2022). In addition, to better understand past changes and effects on the fauna since the ‘Great Acceleration’ of human impacts, we propose palaeoecological studies be undertaken. Such investigations can provide a historical perspective on changing mollusc species diversity in coastal lagoons over the last century or more. Lastly, to fully understand the drivers of recent shifts in mollusc community composition, knowledge of the environmental optima and tolerances for each taxon is required.
Supplementary material
The supplementary material for this article can be found at https://doi.org/10.1017/S0025315423000085.
Acknowledgements
First author NYSM is grateful to the Posgrado en Ciencias del Mar y Limnología, UNAM and the Consejo Nacional de Ciencia y Tecnología de México (CONACyT) for providing a PhD scholarship. We thank all those who participated in the RL1709, RL1802 and RL1805 field sampling surveys. We also thank MSc Yasmin Dávila Jiménez, MSc Mónica Mariel Abarca Avila and MSc Luis Fernando Sauma Castillo for processing and counting samples. We thank BSc Raul Castillo for photographing specimens of Cerithium atratum and Lyonsia sp., and for technical assistance with assigning catalogue numbers, Dra. Patricia Guadarrama for her support and technical assistance in the Laboratory of Marine Ecology and the Coastal zone at the UMDI-Sisal, Faculty of Science, UNAM, and MSc Alfredo Gallardo and MSc Maribel Badillo for technical assistance in the Laboratory of Conservation Biology in the Parque Científico y Tecnológico de Yucatán, UNAM. We also thank MSc Carlos Cruz for the shape file of the map figure. The present research was developed under the framework of CEMIE-Océano (Mexican Centre for Innovation in Ocean Energy), Project FSE-2014-06-249795 financed by CONACYT-SENER-Sustentabilidad Energética. The authors thank the Pedro y Elena Hernández Foundation, the Yucatán Salt Industry (Industria Salinera de Yucatán, ISYSA, S.A. de C.V.), and the Comisión Nacional de Áreas Naturales Protegidas (CONANP) for support during fieldwork. Thanks also to Dra. Edna Naranjo and Dra. Liseth Perez for advice that improved the manuscript. We are grateful to Editor Dr. Natalie Hicks, Dr. Gregory P. Dietl and an anonymous reviewer who greatly contributed to improvement of the manuscript.
Author contributions
NYSM wrote the first draft of the manuscript, identified the molluscs, and prepared tables, figures and plates. VP and MB revised and edited successive drafts of the manuscript. VP participated in interpretation of results. VP and CE contributed to the conception and design of the study, and were responsible for funding acquisition and project administration. NS and CE revised and provided advice on the last draft of the manuscript. All authors contributed to manuscript revision, and have read and agreed to the submitted version.
Financial support
This research was funded by Secretaría de Energía (SENER) and Consejo Nacional de Ciencia y Tecnología (CONACyT) as part of the Centro Mexicano de Innovación en Energía del Océano (CEMIE-Océano, project 249795).