Book contents
- Frontmatter
- Contents
- Preface
- Acknowledgments
- 1 Introduction
- 2 The water in seawater
- 3 Salinity, chlorinity, conductivity, and density
- 4 Major constituents of seawater
- 5 Simple gases
- 6 Salts in solution
- 7 Carbon dioxide
- 8 Nutrients
- 9 Trace metals and other minor elements
- 10 Radioactive clocks
- 11 Organic matter in the sea
- 12 Anoxic marine environments
- 13 Exchanges at the boundaries
- 14 Chemical extraction of useful substances from the sea
- 15 Geochemical history of the oceans
- Appendix A The chemical elements
- Appendix B Symbols, units, and nomenclature
- Appendix C Physical properties of seawater
- Appendix D Gases
- Appendix E Carbon dioxide
- Appendix F Dissociation constants and pH scales
- Appendix G Solubility of calcium carbonate
- Appendix H Effects of pressure
- Appendix I Radioactive decay
- Appendix J Geochemical reservoirs, and some rates
- Appendix K Sound absorption
- Epilogue
- Questions for chapters
- Glossary
- References
- Index
- Miscellaneous end matter
- References
References
Published online by Cambridge University Press: 05 February 2013
- Frontmatter
- Contents
- Preface
- Acknowledgments
- 1 Introduction
- 2 The water in seawater
- 3 Salinity, chlorinity, conductivity, and density
- 4 Major constituents of seawater
- 5 Simple gases
- 6 Salts in solution
- 7 Carbon dioxide
- 8 Nutrients
- 9 Trace metals and other minor elements
- 10 Radioactive clocks
- 11 Organic matter in the sea
- 12 Anoxic marine environments
- 13 Exchanges at the boundaries
- 14 Chemical extraction of useful substances from the sea
- 15 Geochemical history of the oceans
- Appendix A The chemical elements
- Appendix B Symbols, units, and nomenclature
- Appendix C Physical properties of seawater
- Appendix D Gases
- Appendix E Carbon dioxide
- Appendix F Dissociation constants and pH scales
- Appendix G Solubility of calcium carbonate
- Appendix H Effects of pressure
- Appendix I Radioactive decay
- Appendix J Geochemical reservoirs, and some rates
- Appendix K Sound absorption
- Epilogue
- Questions for chapters
- Glossary
- References
- Index
- Miscellaneous end matter
- References
Summary
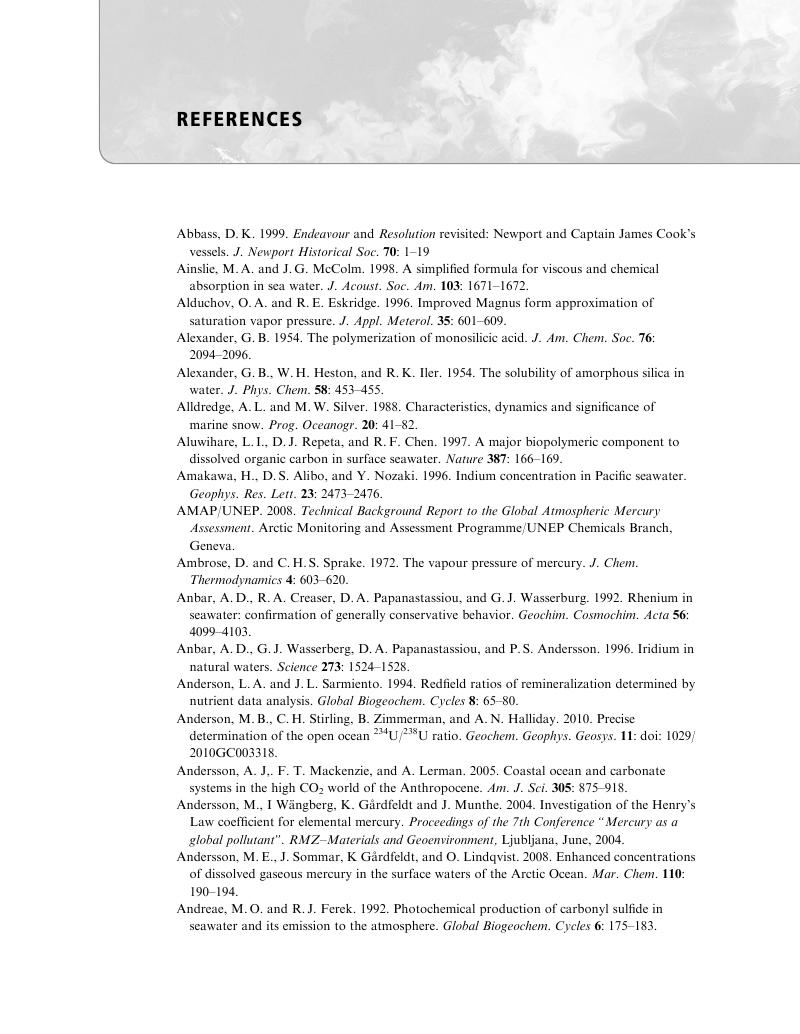
- Type
- Chapter
- Information
- An Introduction to the Chemistry of the Sea , pp. 483 - 515Publisher: Cambridge University PressPrint publication year: 2012