Introduction
Borophagines were a diverse and successful subfamily of canids that thrived throughout North America for nearly 30 million years. The group lived across the continent, and their fossils have been recovered from as far north as Saskatchewan, south into Mexico and Honduras, the Pacific Coast, and more recently along the Atlantic Coast (Wang et al., Reference Wang, Tedford and Taylor1999; Eshelman and Whitmore, Reference Eschelman and Whitmore2008; Wang and Tedford, Reference Wang and Tedford2008; Tseng and Geisler, Reference Tseng and Geisler2016) (Table 1; Fig. 1). The majority of fossil localities where Borophagus is represented indicate environments dominated by relatively open habitats (grassland, savannah, and steppe), although some sites do indicate more extensive vegetation along streams and marshes, or more forested areas on their edges (Cook, Reference Cook1922; Matthew, Reference Matthew1924, Reference Matthew1930; Matthew and Stirton, Reference Matthew and Stirton1930; Meade, Reference Meade1945; Dalquest, Reference Dalquest1969; Bjork, Reference Bjork1970; Hulbert et al., Reference Hulbert, Wallace, Klippel and Parmalee2009). Other vertebrates commonly found with Borophagus include equine and hipparionine horses (including Hipparion, Dinohippus, and Equus) (Cook, Reference Cook1922; Matthew, Reference Matthew1924, Reference Matthew1930; Matthew and Stirton, Reference Matthew and Stirton1930; Meade, Reference Meade1945; Miller and Carranza-Castañeda, Reference Miller and Carranza-Castañeda1998; Hulbert et al., Reference Hulbert, Wallace, Klippel and Parmalee2009), hornless rhinoceros (Aphelops and Teleoceras) (Matthew, Reference Matthew1924, Reference Matthew1930; Matthew and Stirton, Reference Matthew and Stirton1930; Hulbert et al., Reference Hulbert, Wallace, Klippel and Parmalee2009), camels (including Megatylopus, Hemiauchenia, and Camelops) (Cook, Reference Cook1922; Matthew, Reference Matthew1924, Reference Matthew1930; Matthew and Stirton, Reference Matthew and Stirton1930; Meade, Reference Meade1945; Miller and Carranza-Castañeda, Reference Miller and Carranza-Castañeda1998; Hulbert et al., Reference Hulbert, Wallace, Klippel and Parmalee2009), and mastodons (Matthew, Reference Matthew1930; Matthew and Stirton, Reference Matthew and Stirton1930; Meade, Reference Meade1945; Hulbert et al., Reference Hulbert, Wallace, Klippel and Parmalee2009), which have been interpreted as suggesting grass-dominated habitats (Cook, Reference Cook1922; Matthew, Reference Matthew1924, Reference Matthew1930; Matthew and Stirton, Reference Matthew and Stirton1930; Meade, Reference Meade1945; Hulbert et al., Reference Hulbert, Wallace, Klippel and Parmalee2009).

Figure 1. Distribution map of localities for Borophagus specimens. Data sources can be found in Table 1.
Table 1. Previously reported occurrences of Borophagus in North America. Data derived from the MIOMAP/FAUNMAP databases (Carrasco et al., Reference Carrasco, Barnosky, Kraatz and Davis2007; Graham and Lundelius, Reference Graham and Lundelius2010), NOW database (The NOW Community, 2019), and recent publications (Wang et al., Reference Wang, Tedford and Taylor1999; Tseng and Geisler, Reference Tseng and Geisler2016; Wang et al., Reference Wang, White, Balisi, Biewer, Sankey, Garber and Tseng2018). AZ = Arizona; CA = California; CO = Colorado; FL = Florida; ID = Idaho; IN = Indiana; KS = Kansas; NC = North Carolina; NE = Nebraska; NM = New Mexico; NV = Nevada; OK = Oklahoma; OR = Oregon; SC = South Carolina; TN = Tennessee; TX = Texas; WA = Washington.

Appendicular bones are important to understanding the ecology of carnivorans because their predatory behavior has driven the morphological evolution of their limbs (Van Valkenburgh, Reference Van Valkenburgh1987; Samuels et al., Reference Samuels, Meachen and Sakai2013; Martín-Serra et al., Reference Martín-Serra, Figueirido and Palmqvist2016). In general, the anatomy of the forelimbs is very telling of the type of predatory behavior, whereas hind legs give a better indication of speed (Van Valkenburgh, Reference Van Valkenburgh1987; Andersson and Werdelin, Reference Andersson and Werdelin2003; Andersson, Reference Andersson2005; Meachen-Samuels and Van Valkenburgh, Reference Meachen-Samuels and Van Valkenburgh2009; Figueirido and Janis, Reference Figueirido and Janis2011; Fabre et al., Reference Fabre, Cornette, Slater, Argot, Peigné, Goswami and Pouydebat2013; Samuels et al., Reference Samuels, Meachen and Sakai2013; Janis and Figueirido, Reference Janis and Figueirido2014; Figueirido et al., Reference Figueirido, Martín-Serra, Tseng and Janis2015; Martín-Serra et al., Reference Martín-Serra, Figueirido and Palmqvist2016). Postcranial material of Borophagus has been recovered rarely, making their predatory behavior and locomotion controversial, because their appendicular skeleton is extremely different from modern members of Caninae (Dalquest, Reference Dalquest1969; Munthe, Reference Munthe1989; Wang et al., Reference Wang, Tedford and Taylor1999; Figueirido et al., Reference Figueirido, Martín-Serra, Tseng and Janis2015; Martín-Serra et al., Reference Martín-Serra, Figueirido and Palmqvist2016). Borophagus has limb proportions with longer proximal elements and shorter distal elements, leading to the inference that these dogs were not strongly running-adapted (Matthew, Reference Matthew1930; Dalquest, Reference Dalquest1969; Munthe, Reference Munthe1989). Munthe (Reference Munthe1989) hypothesized that the short and robust limbs of Borophagus would have been better adapted for closed habitats where open environments never formed, but with a lack of ecological data at the time, these hypotheses were not able to be assessed. Based on the skeletal and muscular morphology of Borophagus, it was interpreted as flexible and opportunistic in hunting strategies and habitats (Munthe, Reference Munthe1989). Figueirido et al. (Reference Figueirido, Martín-Serra, Tseng and Janis2015) examined the elbow morphology of a wide range of fossil canids, and inferred secondarily derived ambush hunting predatory behavior in Borophagus. The habits of Borophagus in the late Neogene were noted to have contrasted with other canids at the time, which show a general trend toward greater cursorial specialization and pursuit-hunting habits, and further suggests a diversity of (open and closed) habitats were present in North America at the time (Figueirido et al., Reference Figueirido, Martín-Serra, Tseng and Janis2015).
Given the site's age, an Early Pliocene locality located in northeastern Tennessee, the conspicuous absence of Borophagus from the Gray Fossil Site (GFS) was previously noted by multiple researchers (Wang and Tedford, Reference Wang and Tedford2008; Tseng and Geisler, Reference Tseng and Geisler2016). A recently discovered canid humerus (Fig. 2) from GFS is described here as Borophagus. This new occurrence in the heavily forested Appalachian region can provide new insights on its distribution and ecology of the genus.
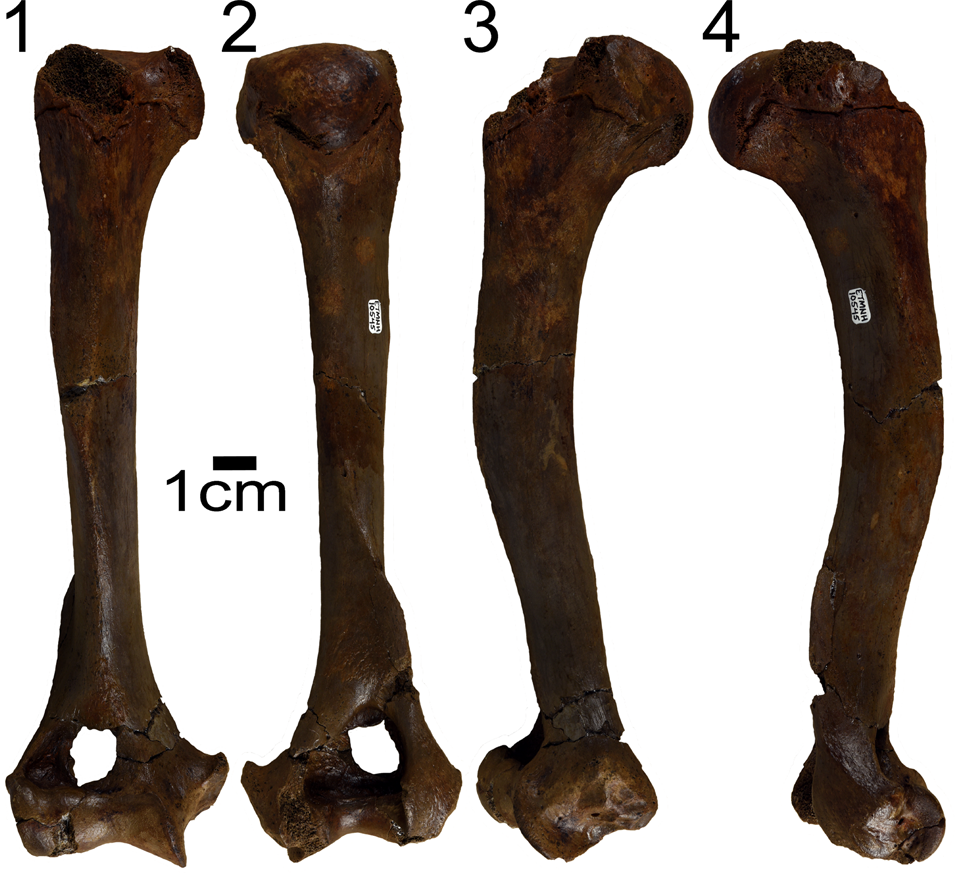
Figure 2. Borophagus sp. (ETMNH 10545) from the Gray Fossil Site, Tennessee. (1) Anterior, (2) posterior, (3) medial, (4) lateral views. Scale bar = 1 cm.
Geologic setting
The Gray Fossil Site in Washington County, Tennessee, is an Early Pliocene (late Hemphillian or early Blancan) fossil locality, which dates to ca. 4.9–4.5 Ma (Samuels et al., Reference Samuels, Bredehoeft and Wallace2018). The site is located in the Appalachian forests of northeastern Tennessee, making it the only pre-Pleistocene vertebrate fossil locality in the Appalachian region of the eastern United States. Studies suggest that the site was formed when an ancient sinkhole collapsed, became a small lake, and then filled with sediment over several thousand years (Wallace and Wang, Reference Wallace and Wang2004; Shunk et al., Reference Shunk, Driese and Clark2006, Reference Shunk, Driese and Dunbar2009). This unique paleontological site records a diverse array of taxa, including a wide variety of flora, invertebrates, fish, amphibians, reptiles, birds, and small and large mammals (Parmalee et al., Reference Parmalee, Klippel, Meylan and Holman2002; Wallace and Wang, Reference Wallace and Wang2004; DeSantis and Wallace, Reference DeSantis and Wallace2008; Boardman and Schubert, Reference Boardman and Schubert2011; Zobaa et al., Reference Zobaa, Zavada, Whitelaw, Shunk and Oboh-Ikuenobe2011; Mead et al., Reference Mead, Schubert, Wallace and Swift2012; Ochoa et al., Reference Ochoa, Whitelaw, Liu and Zavada2012, Reference Ochoa, Zavada, Liu and Farlow2016; Worobiec et al., Reference Worobiec, Liu and Zavada2013; Bourque and Schubert, Reference Bourque and Schubert2015; Jasinski and Moscato, Reference Jasinski and Moscato2017; Jasinski, Reference Jasinski2018; Short et al., Reference Short, Wallace and Emmert2019; Siegert and Hermsen, Reference Siegert and Hermsen2020; Quirk and Hermsen, Reference Quirk and Hermsen2021). Flora and fauna at the site have been interpreted to indicate a relatively closed forested ecosystem (Ochoa et al., Reference Ochoa, Zavada, Liu and Farlow2016; Samuels et al., Reference Samuels, Bredehoeft and Wallace2018; Quirk and Hermsen, Reference Quirk and Hermsen2021). To date, the only carnivorans described from the site are an ailurid, Pristinailurus bristoli Wallace and Wang, Reference Wallace and Wang2004, a meline badger, Arctomeles dimolodontus Wallace and Wang, Reference Wallace and Wang2004, and the earliest record of a wolverine, Gulo sudorus Samuels, Bredehoeft, and Wallace, Reference Samuels, Bredehoeft and Wallace2018. Wallace and Wang (Reference Wallace and Wang2004) also noted the presence of Plionarctos sp., cf. Machairodus sp., and “Canidae” in their faunal list.
Materials and methods
The GFS fossil canid specimen was qualitatively and quantitatively compared to a broad sample of extant and fossil canids (from Samuels et al., Reference Samuels, Meachen and Sakai2013), as well as published descriptions from a variety of sources (Dalquest, Reference Dalquest1969; Munthe, Reference Munthe1989; Wang et al., Reference Wang, Tedford and Taylor1999). Measurements followed Samuels et al. (Reference Samuels, Meachen and Sakai2013) and were taken to the nearest 0.01 mm using digital calipers, these include: total length of the humerus (HuL), anterioposterior diameter (HuAPD), mediolateral diameter (HuMLD), length of pectoral crest (HuPCL), epicondylar breadth of the distal humerus (HuEB), and breadth of the distal articular surface (HuHTL). These six measurements were used to compare the humeral proportions among species, and also to calculate three functional indices (Table 2; Fig. 3) used in a variety of studies (Samuels et al., Reference Samuels, Meachen and Sakai2013). The overall sample includes 20 extant canid species, from 13 genera, and 20 fossil species, with members of all three canid subfamilies represented (complete data are provided in Table 3). Raw measurements and indices were used in linear regressions to make inferences about the limb proportions, locomotor habits, and body size of the canid from GFS using IBM SPSS Statistics 24. Body mass of the GFS specimen was estimated using published regression equations from Anyonge (Reference Anyonge1993) and Figuerido et al. (Reference Figueirido, Pérez-Claros, Hunt and Palmqvist2011).

Figure 3. Linear regression comparisons of humeral proportions among canid species. See Table 3 for species list. (1) Log/ log plot of humerus length against anteroposterior diameter, (2) log/log plot of humerus length against mediolateral diameter, (3) log/log plot of humerus length against deltopectoral crest length, (4) log/log plot of humerus length against epicondylar breadth, (5) log/log plot of humerus length against trochanter length, (6) log/log plot of epicondylar breadth against trochanter length. Extinct taxa are denoted by † symbol.
Table 2. Morphological indices used in the analyses, their definitions, and their inferred functional significance.

Table 3. Humerus measurements (in mm) of Borophagus sp. from the Gray Fossil Site, and species mean values for a comparative sample of extant and fossil canids. Measurements for other canids derived from Samuels et al. (Reference Samuels, Meachen and Sakai2013): Alopex lagopus Linnaeus, Reference Linnaeus1758; Atelocynus microtis (Sclater, 1882); Canis adustus Sundevall, Reference Sundevall1846; Canis latrans Say, Reference Say1823a; Canis mesomelas Hilzheimer, Reference Hilzheimer1906; Cerdocyon thous (Linnaeus, Reference Linnaeus1766); Cuon alpinus (Pallas, Reference Pallas1811); Lycalopex gymnocerus Fischer de Waldheim, Reference Fischer de Waldheim1814; Otocyon megalotis (Desmarest, Reference Desmarest1822); Urocyon cinereoargenteus (Schreber, Reference Schreber1775); Urocyon littoralis (Baird, Reference Baird1857); Vulpes macrotis Merriam, Reference Merriam1888; Vulpes vulpes Linnaeus, Reference Linnaeus1758; Vulpes zerda (Zimmerman, Reference Zimmerman1780); Aelurdon asthenostylus (Henshaw, Reference Henshaw1942); Aelurdon ferox Leidy, Reference Leidy1858; Aelurdon taxoides (Hatcher, Reference Hatcher1893); Archaeocyon stirtoni (Webb, Reference Webb1969b); Archaeocyon leptodus Schlaikjer, Reference Schlaikjer1935; Cynarctus crucidens Barbour and Cook, Reference Barbour and Cook1914; Desmocyon thomsoni (Matthew, Reference Matthew1907); Epicyon saevus (Leidy, Reference Leidy1858); Tomarctus robustus (Green, Reference Green1948); Mesocyon coryphaeus (Cope, Reference Cope1884). Extinct species are denoted by a † symbol.

Repositories and institutional abbreviations
The figured specimen examined in this study is deposited in the East Tennessee State University Museum of Natural History (ETMNH), Tennessee, USA. Additional specimens measured in this study are reposited in the American Museum of Natural History (F:AM), National Museum of Natural History (USNM), Midwestern University (MU), and University of California Museum of Paleontology (UCMP).
Systematic paleontology
Class Mammalia Linnaeus, Reference Linnaeus1758
Order Carnivora Bowdich, Reference Bowdich1821
Suborder Caniformia Kretzoi, Reference Kretzoi1943
Family Canidae Fischer de Waldheim, Reference Fischer de Waldheim1817
Subfamily Borophaginae Simpson, Reference Simpson1945
Genus Borophagus Cope, Reference Cope1892
Type species
Borophagus diversidens Cope, Reference Cope1892 (TMM 40287-10), from Mt. Blanco, Blanco Formation, Crosby County, Texas, U.S.A, by original designation.
Occurrence
Gray Fossil Site, Washington County, Tennessee, Early Pliocene (late Hemphillian or early Blancan age).
Diagnosis
Supratrochlear foramen present, entepicondylar foramen absent, medial condyle enlarged, distal articular surface with mediolaterally broad and proximodistally shallow trochlea, strongly curved diaphysis, and proportions of the element all support assignment to Borophagus.
Description
The GFS humerus (ETMNH 10545) is relatively complete, missing a portion of the greater tubercle and bearing some erosion of the lesser tubercle and posterior surface of the trochlea (Fig. 2). The humeral diaphysis is robust and fairly strongly curved, convex along its anteroposterior axis and minimally convex along the mediolateral axis, and broader anteroposteriorly than mediolaterally. Proximally, the diaphysis is rounded in cross section, but transitions to triangular distally. The proximal epiphysis is not completely fused, suggesting the individual was not fully mature. Due to the incomplete proximal end, we are unable to determine if the greater tubercle projects above the humeral head. The intertubercular groove is relatively broad and shallow. The deltopectoral crest is prominent and elongate, extending more than half-way down the diaphysis. Distally, the humerus is extremely wide compared to its length, in part, due to the enlarged and flanged medial epicondyle. The articular surface is relatively broad and the trochlea is strongly keeled, but the capitulum lacks a spline and the groove between the trochlea and capitulum is shallow. Below the medial epicondyle are four clear facets for muscle attachment, interpreted as the origins of the carpal and digital flexor muscles. The supracondylar ridge is clear, but not particularly expanded. No entepicondylar foramen is present. The radial fossa is relatively small and round, lying directly above the lateral portion of the capitulum. A round and deep coronoid fossa is medial to the radial fossa. The olecranon fossa is roughly triangular in outline, and though the edges are broken, the fossa merges with the coronoid fossa to form a supratrochlear foramen.
Material
ETMNH 10545, right humerus.
Remarks
A number of morphological characteristics of the GFS humerus (ETMNH 10545) facilitate its comparison to previously described humeri of various carnivoran taxa. In canids, the olecranon, coronoid, and radial fossae often fuse to form a supratrochlear foramen; while not observed in all extinct species (Wang and Tedford, Reference Wang and Tedford2008), this feature is characteristic of nearly all canids. In contrast to canids, the supratrochlear foramen is absent in previously described and studied felids, hyaenids, mustelids, procyonids, mephitids, ailurids, ursids, and amphicyonids (Montavon et al., Reference Montavon, Voss and Langley-Hobbs2009). Similarly, ETMNH 10545 does not have an entepicondylar foramen, which is present in many carnivorans, including felids, mustelids, procyonids, mephitids, ailurids, and amphicyonids. The entepicondylar foramen is variably present in ursids (Merriam and Stock, Reference Merriam and Stock1925; Bjork, Reference Bjork1970). Among canids, the entepicondylar foramen is present ancestrally, in taxa from all three canid subfamilies: Hesperocyoninae (Hesperocyon, Mesocyon, and Paraenhydrocyon), Borophaginae (Archaeocyon and Cormocyon), and Caninae (Leptocyon) (Wang, Reference Wang1993; Wang et al., Reference Wang, Tedford and Taylor1999; Tedford et al., Reference Tedford, Wang and Taylor2009; Figueirido et al., Reference Figueirido, Martín-Serra, Tseng and Janis2015). The foramen was lost several times among canids, both within the genus Borophagus and in derived members of the Caninae (both Canini and Vulpini) (Wang et al., Reference Wang, Tedford and Taylor1999; Tedford et al., Reference Tedford, Wang and Taylor2009; Figueirido et al., Reference Figueirido, Martín-Serra, Tseng and Janis2015). The combined presence of a supratrochlear foramen and lack of an entepiconylar foramen indicate the GFS humerus is that of a canid.
In contrast to ETMNH 10545, most members of Caninae (e.g., Canis and Vulpes) have (1) a small medial epicondyle, (2) proximodistally deep and mediolaterally narrow capitulum and trochlea, (3) a deep groove between the capitulum and trochlea, (4) less-curved diaphysis, and (5) proximodistally oriented trochlea (not mediolaterally deflected). Two extant canids studied, Nyctereutes and Speothos, are distinct from other members of the Caninae in a number of ways; both have enlarged medial epicondyles and relatively elongate deltopectoral crest, which reflect their relatively non-cursorial locomotor habits (Samuels et al., Reference Samuels, Meachen and Sakai2013). While Canis (C. ferox Miller and Carranza-Castañeda, Reference Miller and Carranza-Castañeda1998, and C. lepophagus Johnston, Reference Johnston1938), Vulpes (V. stenognathus Savage, Reference Savage1941, and V. velox [Say, Reference Say1823b]), Urocyon (U. galushai Tedford, Wang, and Taylor, Reference Tedford, Wang and Taylor2009), and Eucyon (E. davisi [Merriam, Reference Merriam1911]) are all known from the time period recorded by GFS (Tedford et al., Reference Tedford, Wang and Taylor2009), those taxa do not have humerus morphology or proportions comparable to the GFS canid.
The GFS is an Early Pliocene fossil site and only two species of Borophagus are currently known to have survived into the Pliocene, with both B. diversidens and B. hilli (Johnston, Reference Johnston1939), present in the early Blancan. Given the fact both taxa have broad geographic distributions ranging from the Pacific Northwest to Florida, these are the most likely contenders for the identity of the GFS canid. To date, only two species of Borophagus are actually known to lack an entepicondylar foramen, in all known specimens of B. diversidens (Wang et al., Reference Wang, Tedford and Taylor1999) and some specimens of the late Hemphillian B. secundus (Matthew and Cook, Reference Matthew and Cook1909) (Figueirido et al., Reference Figueirido, Martín-Serra, Tseng and Janis2015), making those species likely candidates for the identity of the GFS canid. However, it is important to note that while B. diversidens and B. secundus have known postcranial material, humeri of B. orc Webb, Reference Webb1969a, B. hilli, and B. dudleyi White, Reference White1941, have not been recovered, and thus those taxa cannot be ruled out. Borophagus dudleyi is also known from the latest Hemphillian of Florida, but is only represented by a single skull, thus it cannot be directly compared to the GFS canid. Borophagus parvus Tedford, Wang, and Taylor, Reference Tedford, Wang and Taylor2009, and B. pugnator (Cook, Reference Cook1922), are also known from the late Hemphillian and were widely distributed, but reported specimens of those taxa possess an entepicondylar foramen (Wang et al., Reference Wang, Tedford and Taylor1999), suggesting they are not the same taxon as the GFS canid.
Results
Age and body size
A study by von Pfeil et al. (Reference von Pfeil and DeCamp2009) examined the epiphyseal plates in modern canids and found that most domestic dogs reach 90% of their adult size by 10 months, with the majority of growth plates fusing between 4–12 months. The proximal humeral epiphysis fully fuses between 10–12 months of age, while the distal humeral epiphysis fuses earlier, between 5–8 months (von Pfeil et al., Reference von Pfeil and DeCamp2009). The distal epiphysis of the humerus from the GFS specimen is fully fused, but the proximal epiphysis is partially fused, suggesting the GFS specimen is most likely between the ages of 8–12 months. A body mass estimate was calculated using published equations; the formula provided by Anyonge (Reference Anyonge1993) yielded a body mass estimate of 74.13kg, while that of Figuerido et al. (Reference Figueirido and Janis2011) produced a body mass estimate of 52.48kg.
Morphometric comparison
The length of the GFS humerus (ETMNH 10545) is greater than most other canids studied, with the exception of the gray wolf (Canis lupus Linnaeus, Reference Linnaeus1758), African hunting dog (Lycaon pictus Griffith, Smith, and Pidgeon, Reference Griffith, Smith and Pidgeon1827), dire wolf (Canis dirus Leidy, Reference Leidy1858), and Armbruster's wolf (Canis armbrusteri) (Table 3). The GFS canid has a very robust shaft (Table 3) and only one other canid studied has a larger diaphyseal diameter; C. dirus. Three other species, C. lupus, C. armbrusteri Gidley, Reference Gidley1913, and the maned wolf (Chrysocyon brachyurus [Illiger, Reference Illiger1815]), have greater mediolateral diameter. The deltopectoral crest length of ETMNH 10545 is relatively large (Table 3), only C. lupus, C. dirus, and C. brachyurus have greater lengths, and each of those taxa has a much longer overall humerus length, meaning the GFS canid has a proportionately longer pectoral crest and thus shoulder moment index (SMI) (Fig. 3). The epicondylar breadth of ETMNH 10545 is the second largest of the canids studied, only behind the much larger C. dirus, but the relative size of the epicondyles (HEB) is much greater than C. dirus and all other canids studied, with exception of the early hesperocyonine Paraenhydrocyon josephi (Cope, Reference Cope1881) (Table 3; Fig. 3). The distal articular surface, including the capitulum and trochlea, is very broad in specimens of Borophagus, greater than all other studied taxa, with exception of the borophagine Epicyon haydeni Leidy, Reference Leidy1858, which is also much larger and relatively broader (compared to humerus length) than in any other canid (Table 3). The shoulder moment index is >0.5 in ETMNH 10545 (Table 3) and in the early canids Hesperocyon gregarius Wortman and Matthew, Reference Wortman and Matthew1899, Paraenhydrocyon josephi, and Phlaocyon leucosteus Matthew, Reference Matthew1899, and the extant Nyctereutes procyonoides (Gray, Reference Gray1834) and Speothos venaticus (Lund, Reference Lund1842). The humeral robustness (HRI) of C. dirus, H. gregarius, and P. josephi is greater than the GFS specimen (Table 3).
Discussion
Recovered postcranial elements of Borophagus are rare, with only 16 specimens having been reported from the fossil record (Dalquest, Reference Dalquest1969; Munthe, Reference Munthe1989; Wang et al., Reference Wang, Tedford and Taylor1999). The addition of the GFS humerus aides in understanding the ecology of the genus, both in terms of locomotion and habitat preference. While the humerus is attributable to the genus Borophagus, there was not enough evidence to assign a species; however, based on the age and morphology of the humerus, the most likely contender is B. diversidens.
The two body mass estimates for Borophagus produced here, 52.48 kg and 74.13 kg, are larger than the average mass of the modern gray wolf (Canis lupus). Figueirido et al. (Reference Figueirido, Martín-Serra, Tseng and Janis2015) provided estimated body mass for several species of Borophagus based on the humerus, with estimated masses of B. parvus = 34.5 kg, B. pugnator = 58.5 kg, and B. secundus = 33.1 kg and 39.1 kg. These estimates are fairly similar, indicating relatively large body mass in Borophagus and supporting inference of predatory specialization for larger taxa (Carbone et al., Reference Carbone, Mace, Roberts and Macdonald1999, Reference Carbone, Teacher and Rowcliffe2007). Cursorial carnivorans have generally gracile limbs characterized by low HRI and have smaller humeral epicondyles, while semifossorial and semiaquatic carnivorans have enlarged humeral epicondyles to allow for larger area for forearm flexor, pronator, and supinator muscles (Samuels et al., Reference Samuels, Meachen and Sakai2013). Humeri in non-cursorial canid taxa are relatively robust, likely as a consequence of ambush predatory behavior, which demands short bursts of speed and extra strength to take down prey (Van Valkenburgh, Reference Van Valkenburgh1985; Munthe, Reference Munthe1989; Anyonge, Reference Anyonge1996; Figueirido et al., Reference Figueirido, Martín-Serra, Tseng and Janis2015; Martín-Serra et al., Reference Martín-Serra, Figueirido and Palmqvist2016). Extant ambush predators have a humeral head that is posteriorly oriented and an anteroposteriorly convex shaft; attributes that suggest the humerus is flexed when taking down prey (Martín-Serra et al., Reference Martín-Serra, Figueirido and Palmqvist2016). Additionally, ambush predators have a large medial epicondyle where the flexor muscles would attach and increase the ability to grasp prey (Martín-Serra et al., Reference Martín-Serra, Figueirido and Palmqvist2016). A shallow trochlea allows supination of the forearm (Andersson, Reference Andersson2005; Figueirido and Janis, Reference Figueirido and Janis2011; Janis and Figueirido, Reference Janis and Figueirido2014; Figueirido et al., Reference Figueirido, Martín-Serra, Tseng and Janis2015; Martín-Serra et al., Reference Martín-Serra, Figueirido and Palmqvist2016), but also indicates long-distance running is not a specialty; instead, animals possessing such features have the ability to perform a variety of tasks (Munthe, Reference Munthe1989; Fabre et al., Reference Fabre, Cornette, Slater, Argot, Peigné, Goswami and Pouydebat2013; Figueirido et al., Reference Figueirido, Martín-Serra, Tseng and Janis2015; Martín-Serra et al., Reference Martín-Serra, Figueirido and Palmqvist2016). The presence of a shallow trochlea with a circular-shaped diaphysis cross section allows movement of the forearm to occur outside the parasagittal plane (Figueirido and Janis, Reference Figueirido and Janis2011; Janis and Figueirido, Reference Janis and Figueirido2014; Figueirido et al., Reference Figueirido, Martín-Serra, Tseng and Janis2015; Martín-Serra et al., Reference Martín-Serra, Figueirido and Palmqvist2016).
The GFS specimen possesses all features of those seen in ambush predator humeri, and given the presence of a heavily forested habitat, for which rapid locomotion was not well suited, Borophagus most likely would have been an ambush predator. Additionally, the presence of Borophagus in Honduras (and now eastern Tennessee), which are parts of North America that never transitioned to open grassland or steppe environments, could suggest Borophagus was adapted to live in both open- and closed-habitat environments (Munthe, Reference Munthe1989).
The shoulder moment index (SMI) of ETMNH 10545 is similar to Hesperocyon gregarious, Paraenhydrocyon josephi, and Phlaocyon leucosteus, indicating a high mechanical advantage across the shoulder joint (Fig. 3). Paraenhydrocyon josephi, an early member of the hesperocyonine subfamily, was adapted for a semi-arboreal lifestyle, while H. gregarious evolved in a forested environment. The humeral robustness indices (HRIs) of C. dirus, H. gregarious, and P. josephi are similar to the GFS specimen (Fig. 3). The relative size of the humeral epicondyles (HEB) in ETMNH 10545 is much greater than all other canids studied, other than P. josephi, and quite distinct from the relatively narrow epicondyles of cursorially adapted pursuit predators (Fig. 3). The large medial epicondyle would have provided for large areas of origin for the wrist and digital flexors, which would have facilitated grasping movements that are typical of ambush hunting predators. Due to the somewhat similar dimensions with the four taxa listed above, it can be concluded the GFS Borophagus was not well adapted for running long distances and most likely was not a pursuit predator. Borophagus may have spent some of its time digging, possibly for refuge from larger competitors (given its size, it needed to feed on prey 50% of its size) (Carbone et al., Reference Carbone, Mace, Roberts and Macdonald1999, Reference Carbone, Teacher and Rowcliffe2007).
Borophagus has been found to inhabit grasslands, transitional, coastal, and now forested habitats. Skeletal and muscular morphology indicate the genus was not adapted for a cursorial lifestyle, which would have been problematic because of their highly cursorial prey. It is thought Borophagus may have been a social animal, and in packs could have hunted larger prey (Wang et al., Reference Wang, White, Balisi, Biewer, Sankey, Garber and Tseng2018). This method may have suited Borophagus in open environments, but specimens found in forested environments, where their skeletal morphology may have been more functionally adapted, may not have needed these pack hunting skills because the forests would have provided sufficient cover for an ambush attack.
Conclusions
The GFS humerus identified as belonging to Borophagus sp. is the first occurrence of the genus in Tennessee and in a heavily forested ecosystem. Weighing between 52–74 kg, the GFS Borophagus is interpreted as adapted to an ambush predatory lifestyle in a closed forested habitat, distinct from the cursorial locomotion other canids living in open habitats. Borophagus would have been one of the largest predators in the GFS forest, alongside saber-toothed cats, and with an abundance of tapirs (Tapirus polkensis Olsen, Reference Olsen1960) and other large herbivores at the site, there certainly would have been enough food resources to support both apex predators.
Acknowledgments
Collection of specimens at the Gray Fossil Site in Tennessee was partially funded through a National Science Foundation Grant (NSF Grant #0958985) to S.C. Wallace and B.W. Schubert. We would like to thank the ETMNH collections managers, A. Nye and B. Compton, who kindly allowed access to specimens in their care. Review and helpful suggestions by X. Wang and A. Atwater improved the quality of this manuscript.
Appendix
Humoral lengths of extant canid specimens studied. Measurement abbreviations include: total length of the humerus (HuL), anterioposterior diameter (HuAPD), mediolateral diameter (HuMLD), length of pectoral crest (HuPCL), breadth of the distal articular surface (HuHTL), and epicondylar breadth of the distal humerus (HuEB).
