Book contents
- The Cambridge Encyclopedia of Child Development
- Child Development
- Copyright page
- Dedication
- Contents
- Contributors
- Editorial preface
- Foreword
- Acknowledgments: External Reviewers
- Introduction Study of child development: an interdisciplinary enterprise
- Part I Theories of development
- Part II Methods in child development research
- Part III Prenatal development and the newborn
- Part IV Perceptual and cognitive development
- Part V Language and communication development
- Part VI Social and emotional development
- Part VII Motor and related development
- Part VIII Postnatal brain development
- Part IX Developmental pathology
- Part X Crossing the borders
- Part XI Speculations about future directions
- Book part
- Author Index
- Subject Index
- Plate Section (PDF Only)
- References
Part III - Prenatal development and the newborn
Published online by Cambridge University Press: 26 October 2017
- The Cambridge Encyclopedia of Child Development
- Child Development
- Copyright page
- Dedication
- Contents
- Contributors
- Editorial preface
- Foreword
- Acknowledgments: External Reviewers
- Introduction Study of child development: an interdisciplinary enterprise
- Part I Theories of development
- Part II Methods in child development research
- Part III Prenatal development and the newborn
- Part IV Perceptual and cognitive development
- Part V Language and communication development
- Part VI Social and emotional development
- Part VII Motor and related development
- Part VIII Postnatal brain development
- Part IX Developmental pathology
- Part X Crossing the borders
- Part XI Speculations about future directions
- Book part
- Author Index
- Subject Index
- Plate Section (PDF Only)
- References
Summary
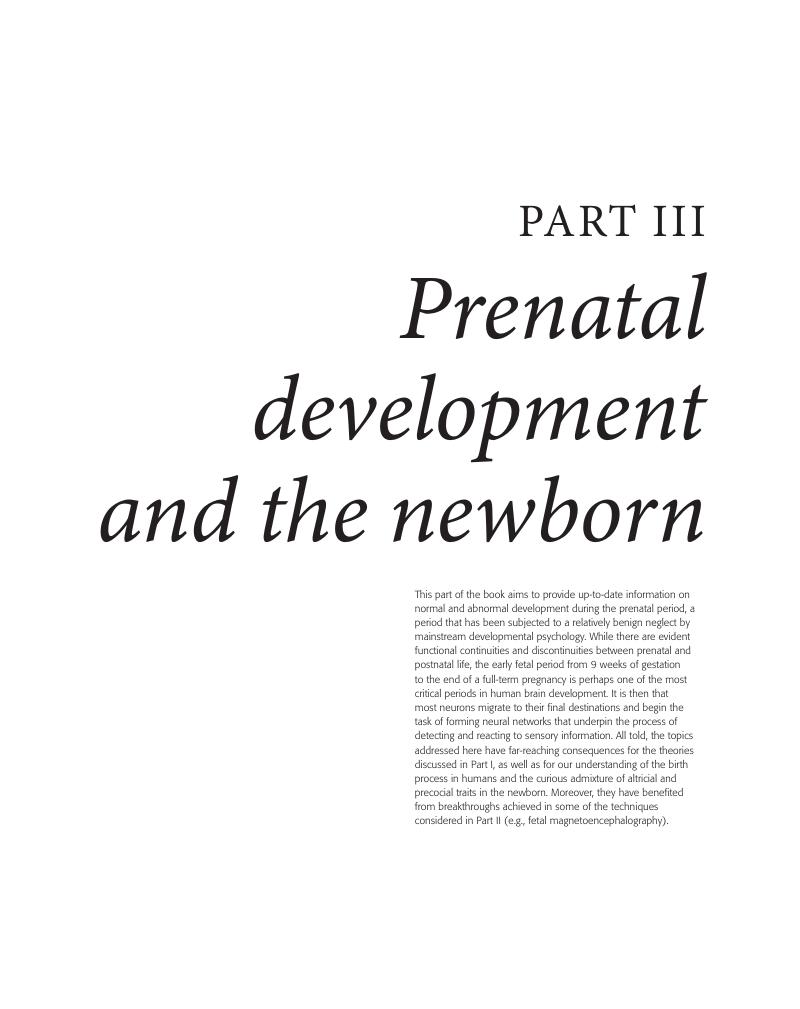
- Type
- Chapter
- Information
- The Cambridge Encyclopedia of Child Development , pp. 211 - 256Publisher: Cambridge University PressPrint publication year: 2017