Book contents
- The Nature of Human Intelligence
- The Nature of Human Intelligence
- Copyright page
- Dedication
- Contents
- Contributors
- Preface
- Chapter 1 Intelligence as Potentiality and Actuality
- Chapter 2 Hereditary Ability: g Is Driven by Experience-Producing Drives
- Chapter 3 Culture, Sex, and Intelligence
- Chapter 4 The Nature of the General Factor of Intelligence
- Chapter 5 Intelligence in Edinburgh, Scotland: Bringing Intelligence to Life
- Chapter 6 Intelligence as Domain-Specific Superior Reproducible Performance
- Chapter 7 Intelligence, Society, and Human Autonomy
- Chapter 8 The Theory of Multiple Intelligences
- Chapter 9 g Theory
- Chapter 10 Puzzled Intelligence
- Chapter 11 A View from the Brain
- Chapter 12 Is Critical Thinking a Better Model of Intelligence?
- Chapter 13 Many Pathways, One Destination
- Chapter 14 My Quest to Understand Human Intelligence
- Chapter 15 Individual Differences at the Top
- Chapter 16 The Intelligence of Nations
- Chapter 17 Intelligences about Things and Intelligences about People
- Chapter 18 Mechanisms of Working Memory Capacity and Fluid Intelligence and Their Common Dependence on Executive Attention
- Chapter 19 Successful Intelligence in Theory, Research, and Practice
- Index
- References
Chapter 10 - Puzzled Intelligence
Looking for Missing Pieces
Published online by Cambridge University Press: 06 January 2018
- The Nature of Human Intelligence
- The Nature of Human Intelligence
- Copyright page
- Dedication
- Contents
- Contributors
- Preface
- Chapter 1 Intelligence as Potentiality and Actuality
- Chapter 2 Hereditary Ability: g Is Driven by Experience-Producing Drives
- Chapter 3 Culture, Sex, and Intelligence
- Chapter 4 The Nature of the General Factor of Intelligence
- Chapter 5 Intelligence in Edinburgh, Scotland: Bringing Intelligence to Life
- Chapter 6 Intelligence as Domain-Specific Superior Reproducible Performance
- Chapter 7 Intelligence, Society, and Human Autonomy
- Chapter 8 The Theory of Multiple Intelligences
- Chapter 9 g Theory
- Chapter 10 Puzzled Intelligence
- Chapter 11 A View from the Brain
- Chapter 12 Is Critical Thinking a Better Model of Intelligence?
- Chapter 13 Many Pathways, One Destination
- Chapter 14 My Quest to Understand Human Intelligence
- Chapter 15 Individual Differences at the Top
- Chapter 16 The Intelligence of Nations
- Chapter 17 Intelligences about Things and Intelligences about People
- Chapter 18 Mechanisms of Working Memory Capacity and Fluid Intelligence and Their Common Dependence on Executive Attention
- Chapter 19 Successful Intelligence in Theory, Research, and Practice
- Index
- References
Summary
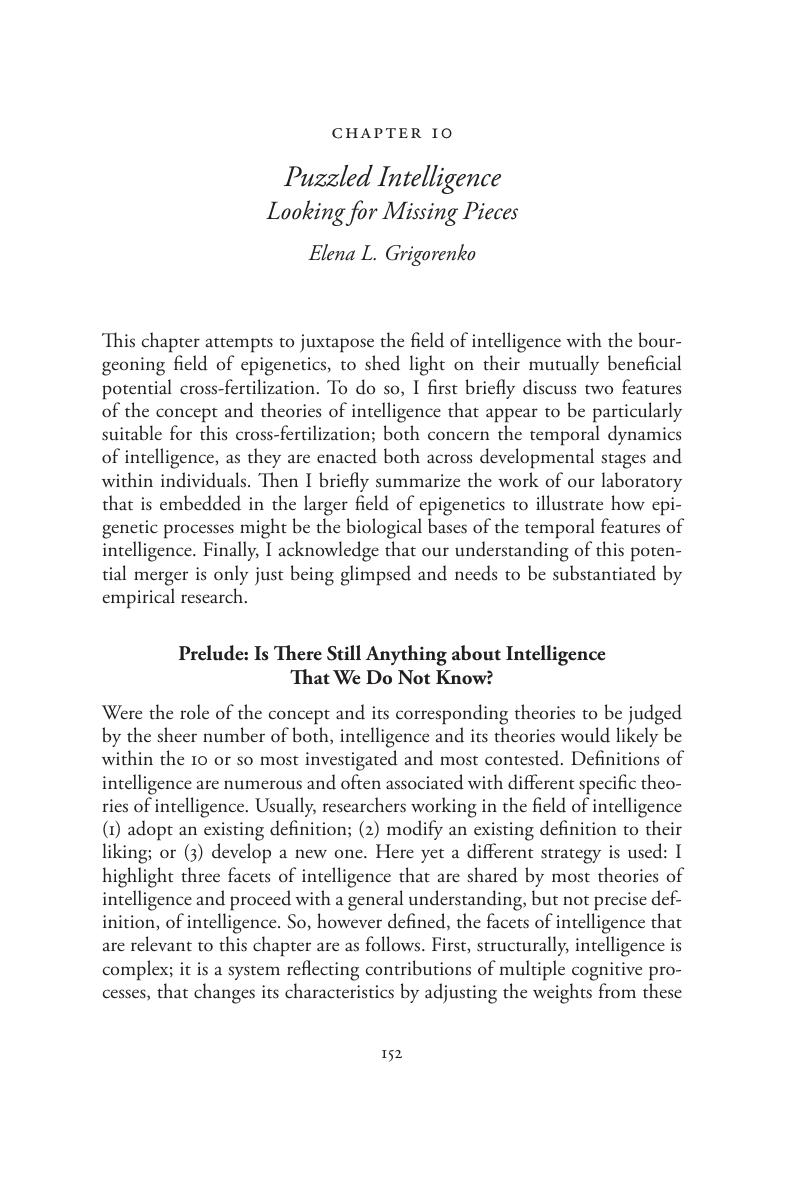
- Type
- Chapter
- Information
- The Nature of Human Intelligence , pp. 152 - 166Publisher: Cambridge University PressPrint publication year: 2018